
Effect of common-rail pressure and natural gas proportion on performance of dual fuel diesel engine
Copyright ⓒ The Korean Society of Marine Engineering
This is an Open Access article distributed under the terms of the Creative Commons Attribution Non-Commercial License (http://creativecommons.org/licenses/by-nc/3.0), which permits unrestricted non-commercial use, distribution, and reproduction in any medium, provided the original work is properly cited.
Abstract
The International Maritime Organization is tightening regulations on air pollution and greenhouse gas emission from ships. One of the resolution strategies for control is the use of the dual fuel diesel engine with natural gas, and it has been widely researched. In this study, a dual fuel diesel engine of 2000cc with common-rail system was adopted and the effects of common-rail pressure and mixing ratio of diesel oil and natural gas on the combustion and emission characteristics of the engine were investigated. A three-step common-rail pressure test was set with increments of 10 MPa and a four-step natural gas ratio test was set under two engine loads. As common-rail pressure was increased by 10 MPa, nitrogen oxides increased by 0.2 g/kWh. As natural gas ratio was increased, the specific energy consumption and nitrogen oxides decreased but carbon monoxide and total hydrocarbon emissions increased. COVIMEP increased with increasing mass fraction of natural gas at low load, which could be attributed to diffusion combustion at low loads. The effect of the COVIMEP value on the stability of operation was negligible.
Keywords:
Dual fuel, Diesel engine, Natural gas, Common-rail pressure, Engine performance1. Introduction
Fuel and combustion methods of internal combustion engines have been changed with rapid changes in climate change and air pollution in the 2000s. The strict Euro 6 and US'10 regulations came into effect in 2014 in Europe and 2010 in the USA. These regulations are concerned with nitrogen oxide (NOx) and particulate matter (PM) emissions. To reduce both NOx and PM emissions simultaneously in diesel engines, Kusaka et al. [1], Ishida et al. [2], Tagai et al. [3], and Saito et al. [4] investigated a premixed charge compression ignition (PCCI) engine fueled with natural gas (NG) and a small amount of gas oil as an ignition source. In dual fuel diesel engines, the combustion method is mainly to burn NG with flame by pilot injection of diesel oil. Many studies have investigated dual fuel diesel engines with NG. Wei and Geng [5] summarized the findings of their study as follows: ① In-cylinder pressure during the compression and initial periods of combustion is slightly lower and ignition delay is longer under the dual fuel mode. ② The dual fuel mode can significantly decrease the NOx, carbon dioxide (CO2) and PM emissions, but hydro-carbon (HC) and carbon monoxide (CO) emissions may increase. ③ The dual fuel mode suffers from low brake thermal efficiency (BTE), especially at low and intermediate loads, while under high engine load conditions, the BTE is similar or slightly higher compared to the normal diesel mode. ④ The coefficient of variation in indicated mean effective pressure (COVIMEP) of the dual fuel mode appears to be generally higher than that of the normal diesel mode and it decreases with increasing engine load. However, these results are common and do not meet the specifications of many diesel engines. Therefore, to commercialize dual fuel diesel engines, experimental results for individual diesel engines are required.
Since early 2000, much research progress has been made in attempts to commercialize the dual fuel diesel engine [6]-[11]. However, the commercialization of dual fuel diesel engines has been achieved in only a fraction of vessels thus far. Therefore it is necessary to test the safety as well as the maximum torque and output according to various factors such as the NG mass fraction, pressure and timing of pilot injection, variable geometry turbo-charger (VGT), and exhaust gas recirculation (EGR). In this study, the combustion and exhaust characteristics of a 2000cc four-cylinder diesel engine were investigated. The effects of pilot injection pressure and NG mixing ratio on combustion characteristics, such as maximum combustion pressure, ignition delay, and premixed combustion, and the effects on exhaust characteristics, such as nitrogen oxides, PM, CO, and THC, were investigated.
2. Experimental Apparatus and Method
2.1 Experimental Apparatus
Table 1 shows specifications of the tested diesel engine and Figure 1 shows a schematic diagram of the experimental equipment. The tested diesel engine (D4EA) is manufactured by Hyundai Motors. Its bore and stroke are 83 mm and 93 mm, respectively. Fuel injection timing, pilot injection, common rail pressure, EGR, and VGT can be controlled. Engine load was controlled using an eddy current type dynamometer (DYTEK-130) manufactured by Hwangwoong Mechatronics Co., Ltd. All factors of the diesel engine were controlled using MotoHawk Control Solutions of Woodward Company on a personal computer in real time. The hardware consists of an electronic control unit (ECM565) and an injector driver (UID800). For the user interface, MotoTune software was used.
Emissions of gases such CO, CO2, NOx, SOx, THC, and O2 were measured using MK9000 manufactured by RBR Ltd. Table 2 shows its specification, and it has a margin of error within 2%. Fuel consumption was measured using an electronic scale, CUW-6200H manufactured by CAS Company. The flow rate of intake air was measured by the differential pressure of a laminar flow meter (LFE-100B) manufactured by Sokken Corporation, and it was calculated in mass units according to the conversion formula. Regarding pressure sensors for the combustion chamber, intake, and exhaust, type 6125C and 4045A5 manufactured by Kistler were used for high pressure and low pressure, respectively. A rotary encoder (E6C2-CWZ) manufactured by OMRON Corporation was used to obtain the trigger signal and an external clock source. To monitor operating conditions of the engine, temperatures of intake and exhaust gases, input and output of cooling water, and fuel and lubricant oil were measured using data acquisition equipment manufactured by the National Instrument Corporation and the software was coded in LabView.
2.2 Experimental Method
All tests were carried out at 2,000 rpm and engine loads of 11.7 kW and 23.0 kW were applied. The pilot injection timing of diesel fuel was 13°BTDC. The common rail pressure and the NG mass fraction were set as shown in Table 3. As the pilot injection period of the diesel fuel was made constant properly, the NG mass fraction became deviated. All experiments were accomplished after a settling period of about 5 min after changing conditions.
Combustion chamber pressure and intake and exhaust pressure were acquired every 0.5° of crank angle per cycle, and 1440 data were acquired. A total of 100 cycles were run and the ensemble average was taken. Various temperature and exhaust emission data were acquired every second, measured for three minutes, and stored in a computer. Fuel consumption was measured by electronic balance for 5 min.
3. Results and Discussion
3.1 Combustion characteristics
Figure 2 shows the variation of combustion pressure (Pcyl) and heat release rate (HRR) in the combustion chamber according to the pressure of the common rail and the NG mass fraction at the low load of 11.7 kW. The solid black line is only for diesel, and the long green dotted line represents 25% of the NG mass fraction, the red dotted line represents 50%, and the blue double dotted line represents 70% or more.
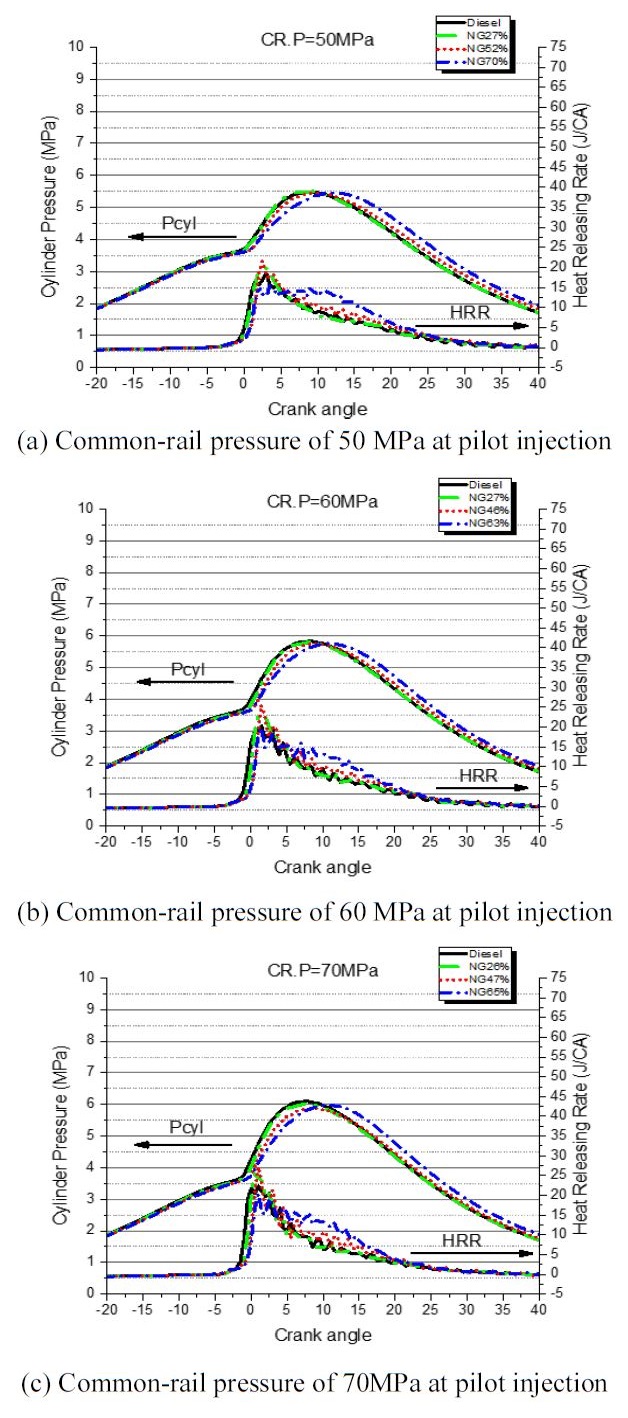
Combustion characteristics with common-rail pressure and mass fraction of NG under an engine load of 11.7kW
As the NG mass fraction was increased, the ignition delay increased gradually because the amount of injected diesel oil decreased. Diffusion combustion was observed when the NG fraction was about 70%. With increasing common rail pressure, the combustion chamber pressure increased by 0.2–0.3 MPa, but there was no significant effect on the difference in combustion characteristics.
Figure 3 shows the variation of combustion pressure and heat release rate at the low load of 23.0 kW. Contrary to the low load, as the NG mass fraction was increased, the amount of premixed combustion increased and the pressure in the combustion chamber increased significantly. No increase in combustion chamber pressure was observed with increasing common rail pressure. This is because the surrounding temperature in the combustion chamber increases with increasing load, and the combustion acceleration phenomenon occurs.
3.2 Cyclic variability
Combustion stability is often expressed by COVIMEP, defined as follows [12]:
(1) |
where σIMEP is the standard deviation of net IMEP and IMEP is the average of the IMEP acquired for 100 cycles.
Figure 4 shows the change in COVIMEP. COVIMEP appeared to increase with increasing NG mass fraction under the low load of 11.7 kW, but the driving characteristics appear to be stable without any increase under the medium load of 23.0 kW. This is related to the increase in diffusion combustion with increasing mass ratio at low load, as shown earlier in Figure 2. Considering that unstable operation corresponds to a value of COVIMEP over 10% [12], there was no unstable operation in this experiment.
3.3 Emission Characteristics
Figure 5 shows the change in NOx emission according to common rail pressure and NG mass fraction. (a) is at the engine load of 11.7 kW and (b) is 23.0 kW. At 11.7 kW, there was no significant decrease in NOx with increasing NG mass fraction, and the lower the common rail pressure, the smaller NOx emissions. NOx increased significantly with increasing NG mass fraction, and the emission was slightly more when the common rail pressure was 70 MPa at 23.0 kW.
Figure 6 shows the change in CO emission according to common rail pressure and NG mass fraction. Under the engine load of 11.7 kW, there was little change in CO emissions due to common rail pressure, and it appeared to increase greatly with increasing mass fraction. This is because NG, which is difficult to burn with low surrounding temperature at low loads, affects the production of CO. Under 23.0 kW, no significant change was observed in CO emissions with increasing mass fraction, and it increased with increasing common rail pressure. CO is considered to be produced more in richer areas in general.
Figure 7 shows the change in THC emission according to common rail pressure and NG mass fraction. Regardless of the load, THC emission did not vary according to the common rail pressure, and it increased with increasing mass fraction. As the load was increased, THC decreased significantly. This may be due to the high probability of incomplete combustion at low loads.
Figure 8 shows the change of CO2 emission according to common rail pressure and NG mass fraction. Under 11.7 kW, the higher the common rail pressure, the larger the CO2 emissions. Similar values were observed under 23.0 kW regardless of the common rail pressure. As the NG mass fraction decreased, the CO2 emissions decreased, regardless of load, with a maximum reduction of 30%. Thus, NG is expected to have an excellent effect on reducing carbon dioxide, a greenhouse gas.
4. Conclusion
Combustion and exhaust characteristics of a 2000cc four-cylinder diesel engine were investigated to understand the effects of pilot injection pressure and NG mixing ratio on combustion. The main conclusions can be summarized is as follows:
- 1) As the NG mass fraction increased, diffusion combustion was observed at 11.7 kW, and at 23.0 kW, the amount of premixed combustion increased and the pressure in the combustion chamber increased significantly.
- 2) COVIMEP increased as the NG mass fraction increased at a low load of 11.7 kW, but stable driving characteristics were observed without any increase at a medium load of 23.0 kW. This is related to the characteristic of diffusion combustion at low loads.
- 3) There was no significant decrease in NOx with increasing NG mass fraction at 11.7 kW, but NOx markedly increased with increasing NG mass fraction at 23.0 kW
- 4) THC emission did not vary with common rail pressure, but it exhibited an increasing trend with increasing mass fraction.
- 5) As the NG mass fraction decreased, CO2 emissions decreased, regardless of load.
Acknowledgments
This work was supported by a Research Grant of Pukyong National University (2019).
Author Contributions
Conceptualization, M. T. Zin; methodology, M. T. Zin and J. M. Chen; Software, S. H. Jung; Formal Analysis, author’s name; Investigation, M. T. Zin and J. M. Chen; Resources, M. T. Zin; Data curation M. T. Zin and J. M. Chen; Writing-Original Draft Preparation, M. T. Zin; Writing-Review & Editing, J. M. Chen; Visualization, J. M. Chen; Supervision, S. H. Jung; Project Administration, S. H. Jung; Funding Acquisition, S. H. Jung.
References
- J. Kusaka, Y. Daisho, R. Kihara, and T. Saito, “Combustion and exhaust gas emission characteristics of a diesel engine dual-fueled with natural gas,” Proceeding of the 4th International Symposium COMODIA, pp. 555-560, 1998. Available: https://www.jsme.or.jp/esd/COMODIA-Procs/Data/004/C98_P555.pdf, .
-
M. Ishida, T. Tagai, and H. Ueki, “Effect of EGR and preheating on natural gas combustion assisted with gas-oil in a diesel engine,” JSME International Journal, Series B, vol. 46, no. 1, pp. 124-130, 2003.
[https://doi.org/10.1299/jsmeb.46.124]
-
T. Tagai, M. Ishida, H. Ueki, and T. Watanabe, “Effect of equivalence ratio and temperature of CNG premixture on knock limit in a dual fueled diesel engine,” SAE 2003 Transactions, Journal of Fuels and Lubricants, vol. 112, pp. 1807-1816, 2003. Available: https://www.jstor.org/stable/44742399, .
[https://doi.org/10.4271/2003-01-1934]
-
H. Saito, T. Sakurai, T. Sakonji, T. Hirashima, and K. Kanno, “Study on lean burn gas engine using pilot oil as the ignition source, SAE Transactions, vol. 110, pp. 1-7, 2001.
[https://doi.org/10.4271/2001-01-0143]
-
L. Wei and P. Geng, “A review on natural gas/diesel dual fuel combustion, emissions, and performance, Fuel Processing Technology, vol. 142, pp. 264-278, 2016.
[https://doi.org/10.1016/j.fuproc.2015.09.018]
-
C. Poompipatpong and K. Cheenkachorn, “A modified diesel engine for natural gas operation: Performance and emission tests,” Energy, vol. 36, no. 12, pp. 6862-6866, 2011.
[https://doi.org/10.1016/j.energy.2011.10.009]
-
K. Cheenkachorn, C. Poompipatpong, and G. H. Choi, “Performance and emissions of a heavy-duty diesel engine fuelled with diesel and LNG,” Energy, vol. 53, no. 1, pp. 52-57, 2013.
[https://doi.org/10.1016/j.energy.2013.02.027]
-
T. Korakianitis, A. M. Namasivayam, and R. J. Crookes, “Natural-gas fueled spark-ignition (SI) and compression-ignition (CI) engine performance and emissions,” Progress in Energy and Combustion Science, vol. 37, no. 1, pp. 89-112, 2011.
[https://doi.org/10.1016/j.pecs.2010.04.002]
- J. Sim et al., “A study on improvement of diesel substitution ratio through changing system of mechanical diesel engine to diesel-LNG dual fuel engine,” Proceedings of KASE annual autumn conference, pp. 199-205, 2012.
- M. D. Eom et al., “The evaluation on smoke reduction by natural gas dual fuel engine for city bus,” Journal of Korea Air Pollution Research Association, vol. 13, no. 3, pp. 215-220, 1997.
-
M. H. Park, “A development of converting technology for the marine gasoline/CNG Bi-fuel engine,” The Korean Society of Marine Engineering,” vol. 34, no. 5, pp. 632-637, 2010.
[https://doi.org/10.5916/jkosme.2010.34.5.632]
- John Heywood, Internal Combustion Engine Fundamentals, New York, McGraw-Hill Book Company, 1988.