
Characteristics of dissolved gases separated from water mixed with exhalation gases without using a compressor
Copyright © The Korean Society of Marine Engineering
This is an Open Access article distributed under the terms of the Creative Commons Attribution Non-Commercial License (http://creativecommons.org/licenses/by-nc/3.0), which permits unrestricted non-commercial use, distribution, and reproduction in any medium, provided the original work is properly cited.
Abstract
It is possible for humans to breathe underwater using dissolved oxygen. However, unlike fish, humans need large amounts of oxygen to breathe underwater. Water generally contains small amounts of dissolved oxygen. To get enough dissolved oxygen from water, great volumes of it should be supplied into a separation device. If exhalation gases are used, the amounts of water supplied into the membrane can be decreased. However, the characteristics of exhalation gases after passage through the separation device need to be investigated. To reuse the exhalation gases, the concentration of carbon dioxide should be decreased. A compressor is needed to supply the exhalation gases because of the high pressure generated in the membrane inlet. However, compressors require a lot of power and are heavy, so it is not proper to get the portable separation device. A system without the compressor is needed. If the pressure of the position mixed from the exhalation is less than atmosphere, the compressor is not needed.
In this thesis, characteristics of the gases which are mixed with exhalation gases and separated from water after passing the membrane are investigated. The compositions of carbon dioxide, oxygen, and nitrogen are measured with the gas chromatography. The effects of water and exhalation gas flow rates on characteristics of gases separated from water after the membrane are showed.
Keywords:
Exhalation gases, Without compressor, Underwater, Separation, Mixed1. Introduction
It is possible for humans to breathe underwater using the dissolved oxygen. By observing an insect drowned underwater, it is reported that there is possibility to take use of dissolved oxygen [1]. There are furs on the surface of the insect which make it possible to breathe underwater. To investigate these characteristics, porous and hydrophobic materials are used to make a rectangular box, which has an oxygen sensor at the top and its inside volume is removed to include gases and is drowned underwater. Water without oxygen and with rich oxygen is supplied to the storage tank. At that time, oxygen concentration is measured to know if the dissolved oxygen is moved from box into water or from water to the box. When water without dissolved oxygen is supplied, oxygen concentration of inside of the box is decreased. It means that oxygen of inside box is moved to water. When water with rich oxygen is supplied, the concentration of inside of the box is increased. It means that oxygen contained in water is transferred to inside of the box. If there is a cell consuming oxygen inside of the box, oxygen concentration of the box is decreased when the box with the general material is used. But when the box with porous and hydrophobic material is used, the concentration of inside of the box is firstly decreased and finally saturated. It means that when the box with porous and hydrophobic material is used, firstly the concentration of inside of the box is decreased with consuming oxygen inside of the box and then saturated with being supplied by dissolved oxygen contained in water. A human can be regarded as component consuming oxygen. So, if there is the box with great surface area which is characterized by porous and hydrophobic material, a human can breathe underwater. Size of the box needs to be decreased for a human to carry the box. As another case, it is reported that furs on the surface of the insect make it possible to make bubble between water and the body of one [2]. Bubbles make it possible for an insect to survive underwater. The diameter of a fur, the distance between furs and the contact angle of one have effects on surviving bubble underwater. The deeper it is, the more pressure it has. At deep water, because of high water pressure, interface surface between water and body surface of the insect is increased and moving of oxygen is increased. But more water pressure makes bubble broken out underwater. So, proper depth is needed for an insect to survive underwater. At shallow water, the surface area between water and surface of the body is decreased. Moving of oxygen between water and bubble is decreased and it makes it difficult for an insect to survive underwater. The insect with limited diameter of a fur, inter length between furs, contact angle of a fur has minimum and maximum depth.
The structure of a fish gill is also investigated to characterize the separation of dissolved oxygen [3]. It is reported that a fish gill dimension has a proper ratio. To increase the amounts of separation of dissolved oxygen, a separation device with high surface area needs to be used [4]. Also, magnetic materials are used to separate more dissolved oxygen from water [5].
In this thesis, when exhalation gases are recirculated to inlet water, characteristic of the gases separated from water after the membrane module is investigated [6]. The compositions of carbon dioxide, oxygen and nitrogen are measured with the gas chromatography. The effects of water and exhalation gas flow rates on characteristics of gases separated from water after the membrane module are showed.
2. Experimental
The structure of a fish gill is shown in Figure 1. The gills of a fish are placed near the eyes, as shown in Figure 1 (a). The gill of a fish contains a gill arch and gill filaments as showed in Figure 1 (b). The oxygen poor blood is out of the fish body and is changed into the oxygen rich blood through the gill of a fish as showed in Figure 1 (c). The oxygen poor blood has low concentration of oxygen whereas water contains rich oxygen. The oxygen poor blood is changed into oxygen rich blood due to the oxygen gradient, passing through the gill of a fish as shown in Figure 1 (d). The oxygen transferring rates are proportional to the surface area of the gill of a fish.
As shown in Figure 2, porous and hydrophobic material needs to be used to separate dissolved gases from water. There is water in the left side, whereas there are separated gases in the right side. The amounts of separation of dissolved gases are increased if pressure is decreased in the right side. Water contains a little amount of dissolved oxygen, so more contact area is requested to separate more dissolved gases from water. But large amounts of dissolved gases need a larger contact area. It requires the separation device to have a large volume. So the surface area per unit volume needs to be increased. In Table 1, the surface area per a volume is listed. The hollow fiber type membrane has more packing density than the flat sheet type membrane and has advantage for the portable device. The hollow fiber used has 300 m of outer diameter and 200 μmm of inner diameter with polypropylene characteristics.
The outline for the experimental device shown in Figure 3 represents the membrane module, water pump, gas flow meter, sampling bag and exhalation gases. A membrane module from Liqui-Cel was used with 8.1 m2 of surface area. The water pump is positioned after the membrane module and soaks inlet water. Before the membrane module, the water tank, water flow meter and exhalation gas mixer are positioned. Using the vacuum pump, dissolved gases including dissolved oxygen are separated from water. Separated gases are moved and stored to the sampling bag. Compositions of sampled gases are measured with a gas chromatograph (GC). Firstly, synthesized gases based on exhalation gases for a human are mixed to the inlet water from 1 LPM to 5 LPM by 1 LPM. Furthermore, real-time exhalation gases are supplied to the mixer port in inlet water and dissolved gases are separated from water using both a vacuum pump and the membrane module. Lastly, when 5 L of sampling bag containing exhalation gases from a human is connected to inlet of the separation system, the amounts of dissolved gases separated from water are measured. The experimental devices are shown in Figure 4. The pressure measured before the membrane module is represented in Figure 5 and has 87.1 kPa less than atmosphere state. Supplied water is measured as 37 LPM.
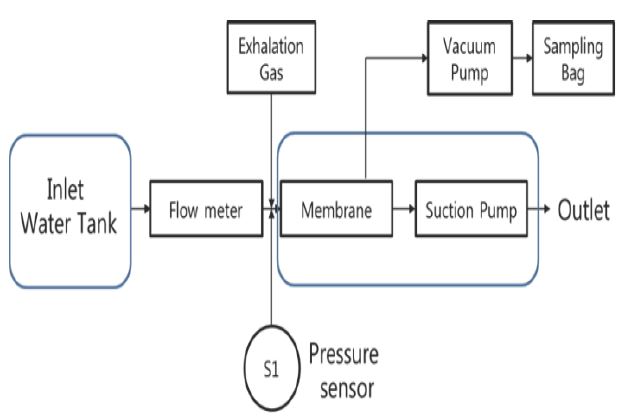
Outline for analyzing gases separated from water mixed with exhalation gases using a separation system lacking a compressor
3. Results and discussions
The pore distribution is measured to characterize a polypropylene hollow fiber used in membrane module as shown in Figure 6. The x-axis represents a pore diameter and y-axis means a volume variation of a hollow fiber. A high proportion is measured near at 0.1 μm pore diameter. A mercury porosimeter from Quantachrome Instruments were used to investigate the pore distribution. At first, it weighs mass of a material and the pore characteristics of a material are investigated during pressing mercury into a material with pressure. The operation of all mercury porosimeters is based upon the physical principle that a nonreactive, non-wetting liquid will not penetrate fine pores until sufficient pressure is applied to force its entry.
Compositions of a synthesized exhalation are shown in Figure 7 and compose of oxygen, nitrogen, and carbon dioxide. 17.25 % of oxygen, 78.9 % of nitrogen, and 4.35 % of carbon dioxide are measured. Figure 8 shows compositions of separated gases when 1 LPM of synthesized gas is mixed to inlet water. The amounts of carbon dioxide is decreased to 0.5% and one of oxygen increased to 22.38%. When 2 LPM of exhalation gas is recirculated to inlet water, as shown in Figure 9, the amounts of carbon dioxide are decreased to 0.8% and have a little higher concentration than one of 1 LPM synthesized exhalation gas. Figure 10, Figure 11, and Figure 12 show the compositions of carbon dioxide, oxygen, and nitrogen separated from the water after passing through the membrane module when 3 LPM, 4 LPM, and 5 LPM of synthesized gas were mixed with the inlet water. The amounts of carbon dioxide are increased to 0.9%, 1.2%, and 1.5% with mixed exhalation gases but have less than one of original synthesized exhalation gas. These results mean that carbon dioxides were dissolved in water and the amounts of carbon dioxides contained in separated gases were decreased. The amounts of oxygen are decreased to 20.49%, 19.76%, and 19.16% and have more than one of original synthesized exhalation gas, so these results can be applied to underwater breathing device. These results means that some oxygen dissolved in water were separated through the membrane module.
Figure 13 shows compositions of separated gases from the membrane module when real-time exhalation gases are used. 0.96% of carbon dioxide and 20.58% of oxygen are measured. The amounts of separated oxygen have similar value to one of an air. Figure 14 represents compositions of separated gas when sampled bag which contains the exhalation gas is mixed to inlet water. 0.95% of carbon dioxide and 20.25% of carbon dioxide are measured. The concentration of oxygen with real-time exhalation gas is a little more than one with a 5 L sampling bag, but represents roughly similar value to one with a 5 L sampling bag. For the actual application, the sampling bag is efficient because the exhalation is separated from mixing part in the water inlet and exhalation resistance is minimized.
4. Conclusions
If dissolved oxygen contained in water is properly used, it is possible for a human to breathe underwater. But water has small amounts of oxygen, if dissolved oxygen is separated from water and used to breathe underwater, the large separating device with high surface area is needed. Generally, exhalation gases have some oxygen and carbon dioxide and nitrogen. If the concentration of carbon dioxide is decreased less than some safely level, it is useful to make use of the exhalation gases. The compressor is generally needed to mix the exhalation gases but it also makes the size, weight, and power of the separation device higher. If the device using dissolved oxygen separated from water is effectively used as a portable type of underwater breathing apparatus, the size and the power consumption of that device need to be decreased.
In this thesis, the pressure at the position which the exhalation gas is mixed with inlet water before the membrane module was lowered to below atmosphere pressure. Hence, without a compressor, the exhalation gases were introduced to the inlet water and separated from membrane module using a vacuum pump. The exhalation gases were mixed to inlet water and passed through the membrane module. Water flowed in the shell side of hollow fibers and exited, and dissolved gases were separated in the lumen side of fibers. Compositions of separated gases were measured using the GC. Synthesized gases based on the exhalation gas for a human were generated, increased and mixed to inlet water from 1 LPM to 5 LPM by 1 LPM. When the amounts of synthesized exhalation gas were increased, concentration of carbon dioxide increased and that of oxygen slightly decreased. When real-time exhalation gas was mixed with inlet water, concentration of carbon dioxide was 0.96% and that of oxygen showed 20.58%. When 5 L of sampling bag was used, 0.95% of carbon dioxide and 20.25% of oxygen were measured. It is meaningful that the amounts of carbon dioxide are less than 1%. The concentration of oxygen was roughly similar to that of air. Hence, in the future, these results are expected to apply to the development of underwater breathing device technology using dissolved oxygen.
Acknowledgments
This research was supported by the research program (NK200H) of Korea Institute of Machinery and Materials funded by the National Research Council of Science and Technology.
This paper is extended and updated from the short version that appeared in the Proceedings of the International Symposium on Marine Engineering and Technology (ISMT 2016), held at Korea Maritime and Ocean University, Busan, Korea, November 3–4, 2016.
References
-
N. J. Shirtcliffe, G. McHale, M. I. Newton, C. C. Perry, and F. B. Pyatt, “Plastron properties of a superhydrophobic surface”, Applied Physics Letters, 89(10), p104106-1-104106-2, (2006).
[https://doi.org/10.1063/1.2347266]
-
M. R. Flynn, and J. W. M. Bush, “Underwater breathingthe mechanics of plastron respiration”, Journal of Fluid Mechanics, 608, p275-296, (2008).
[https://doi.org/10.1017/S0022112008002048]
-
K. H. Park, W. J. Kim, and H. Y. Kim, “Optimal lamellar arrangement in fish gills”, PNAS, 111(22), p8067-8070, (2014).
[https://doi.org/10.1073/pnas.1403621111]
- P. W. Heo, and I. S. Park, “Separation characteristics of dissolved gases from water Using a polypropylene hollow fiber membrane module with high surface area”, World Academy of Science, Engineering and Technology, 8(7), p1266-1269, (2014).
-
Velianti, S. B. Park, and P. W. Heo, “The enhancement of oxygen separation from the air and water using poly(vinylidene fluoride) membrane modified with superparamagnetic particles”, Journal of Membrane Science, 466, p274-280, (2014).
[https://doi.org/10.1016/j.memsci.2014.04.043]
- P. W. Heo, “Characteristics of dissolved gases separated from water mixed by exhalation gases without the compressor”, International symposium on Marine Engineering and Technology, p54, (2016).