
Fabrication of nickel nanoparticles-embedded carbon particles by solution plasma in waste vegetable oil

Copyright © The Korean Society of Marine Engineering
This is an Open Access article distributed under the terms of the Creative Commons Attribution Non-Commercial License (http://creativecommons.org/licenses/by-nc/3.0), which permits unrestricted non-commercial use, distribution, and reproduction in any medium, provided the original work is properly cited.
Abstract
Solution plasma is a unique method which provides a direct discharge in solutions. It is one of the promising techniques for various applications including the synthesis of metallic/non-metallic nanomaterials, decomposition of organic compounds, and the removal of microorganism. In the context of nanomaterial syntheses, solution plasma has been utilized to produce carbon nanoparticles and metallic-carbon nanoparticle systems. The main purpose of this study was to synthesize nickel nanoparticles embedded in a matrix of carbon particles by solution plasma in one-step using waste vegetable oil as the carbon source. The experimental setup was done by simply connecting a bipolar pulsed power generator to nickel electrodes, which were submerged in the waste vegetable oil. Black powders of the nickel nanoparticles-embedded carbon (NiNPs/Carbon) particles were successfully obtained after discharging for 90 min. The morphology of the synthesized NiNPs/Carbon was investigated by a scanning electron microscope, which revealed a good dispersion of NiNPs in the carbon-particle matrix. The X-ray diffraction of NiNPs/Carbon clearly showed the co-existence of crystalline Ni nanostructures and amorphous carbon. The crystallite size of NiNPs (through the Ni (111) diffraction plane), as calculated by the Scherrer equation was found to be 64 nm. In addition, the catalytic activity of NiNPs/Carbon was evaluated by cyclic voltammetry in an acid solution. It was found that NiNPs/Carbon did not show a significant catalytic activity in the acid solution. Although this work might not be helpful in enhancing the activity of the fuel cell catalysts, it is expected to find application in other processes such as the CO conversion (by oxidation) and cyclization of organic compounds.
Keywords:
Ni/Carbon nanoparticle, Solution plasma, Catalyst, Waste vegetable oil1. Introduction
Solution plasma is a relatively new process and is considered to be an efficient method for producing various nanomaterials such as metallic nanoparticles, carbon nanoparticles, metallic nanoparticles-nanoporous material systems, and metallic nanoparticles-carbon material systems [1]-[7]. Recently, there have been reports of using solution plasma for the preparation of carbon nanoparticles by a one-step discharge in organic solvents. The formation of carbon can be explained by the dissociation and recombination of active carbon species from the carbon precursor during discharge [6]-[8]. Different organic solvents (carbon precursor) produce carbon particles with different functionalities. A mixture of pyrrole and dodecane can be used as a nitrogen-containing carbon source to obtain nitrogen-doped carbon nanoparticles with good catalytic activity and high durability for fuel cell applications [8].
Ni is a non-precious metal and is used as a catalyst in various processes such as the CO conversion, cyclization, and cycloaddition of organic compounds [9][10]. Ni nanoparticles (NiNPs) deposited on carbon supports have emerged as promising catalysts because of their excellent catalytic performance due to their large surface areas. NiNPs/Carbon has been reported to show catalytic activity towards oxygen reduction in basic solutions [11]. NiNPs/Carbon has also been reported to be able to catalyze the oxidation of CO and hydrocarbons at low temperatures [11].
In this study, the NiNPs-embedded carbon (NiNPs/Carbon) particles were prepared by a one-step synthesis procedure using solution plasma [12]. NiNPs were formed by the sputtering effect at Ni electrodes and the carbon particles were formed by the dissociation and recombination of carbon species in waste vegetable oil during the solution plasma discharge. Several characterization techniques such as scanning electron microscopy (SEM), energy-dispersive X-ray spectroscopy (EDX), and X-ray diffraction (XRD) were used to investigate the morphology, elemental composition, and crystal structure of NiNPs/Carbon, respectively. In addition, cyclic voltammetry (CV) was carried out to evaluate the catalytic activity of NiNPs/Carbon towards oxygen reduction.
2.Methodology
2.1 Preparation of NiNPs/Carbon particles by solution plasma
The solution plasma-assisted synthesis of NiNPs/Carbon was carried out according to the procedure reported by Kim et al. [8]. A 100 mL glass beaker was used as the reactor into which two Ni electrodes with a diameter of 1 mm were introduced. Waste vegetable oil obtained from a school canteen was used as the carbon precursor. The waste vegetable oil was poured into the reactor. The oil was discharged by applying a high pulsed voltage through the Ni electrodes using a bipolar pulsed power generator (Pekuris, MPP-HV02, Japan) under vigorous stirring. The pulse width, frequency, electrode gap, and discharge time were set at 1 μs, 15 kHz, 0.5 mm, and 90 min, respectively. A black solid product was obtained, which was then filtered and washed several times with hexane and ethanol. The washed product was dried in an oven at 100 °C for 12 h. Subsequently, it was heat-treated at 900 °C for 30 min under the flow of N2 (500 sccm). The purpose of this heat treatment was to rearrange the carbon structure, and thus increase the conductivity of the carbon-particle matrix.
2.2 Characterizations
The SEM and EDX (Hitachi TM3030Plus) analyses were carried out at an acceleration voltage of 15.0 kV to examine the microstructure, shape, size, and elemental composition of NiNPs/Carbon. The crystal structure of NiNPs/Carbon was examined by its XRD pattern, which was collected by Rigaku SmartLab at 40 kV and 30 mA. The XRD analysis was carried out by using a Cu Kα radiation (1.54 Å) over a scan range (2θ) of 5–90° with a scanning step of 0.01 and a scanning speed of 4°/min.
The catalytic activity of NiNPs/Carbon was electrochemically evaluated by CV. The CV measurements were carried out in a three-electrode cell (μAUTOLABIII/FRA2) using 0.1 M H2SO4 as the electrolyte. The electrolyte was saturated with O2 by purging O2 gas into the cell. A glassy carbon (GC) disk (3 mm in diameter) was used as the working electrode, a platinum electrode was used as the counter electrode, and an Ag/AgCl (saturated KCl) electrode was used the reference electrode. The CV scans were performed in the voltage range of -0.2 - 1.4 V at a rate of 50 mV/s.
3. Results and discussion
3.1 Morphology and elemental analysis by SEM and EDX
After the completion of all the processing steps (solution plasma discharge through heat treatment), approximately 100 mg of NiNPs/Carbon was obtained from 100 mL of waste vegetable oil, which acted as the carbon precursor. NiNPs and carbon were formed by the sputtering of the Ni electrodes and the dissociation and recombination of carbon species from the waste vegetable oil during the solution plasma discharge, respectively [6]-[8]. The SEM images of NiNPs/Carbon are shown in Figure 1. The images reveal that NiNPs were successfully embedded in the carbon-particle matrix with good dispersion. NiNPs had a spherical morphology and were dispersed on carbon particles with a mean size of about 80 μm. The carbon particles showed a chain-like morphology. This can be attributed to their Brownian motion during the solution plasma processing. The chain-like carbon particles were interconnected in many directions because of the collision of individual particles. This caused the agglomeration of these particles, which then resulted in the formation of a three-dimensional network [6].
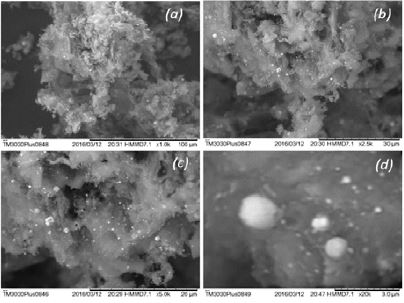
Typical SEM images of NiNPs/Carbon prepared by solution plasma in waste vegetable oil at various magnifications: (a) ×1000, (b) ×2500, (c) ×5000, and (d) ×20000.
Figure 2 (b, c, e, and f), shows the elemental analysis of NiNPs/Carbon. The EDX spectrum revealed the presence of C, Ni, O, and P. Ni was present on the carbon particles. A C peak was also detected. The O peak is attributed to the oxygen present in the oil, while the P peak is attributed to the phospholipid groups of the oil. The distribution of NiNPs was confirmed by the EDX mapping shown in Figure 2 (g–j). As can be seen, NiNPs were highly dispersed on the carbon surface. Hence, NiNPs/Carbon had a large surface area.
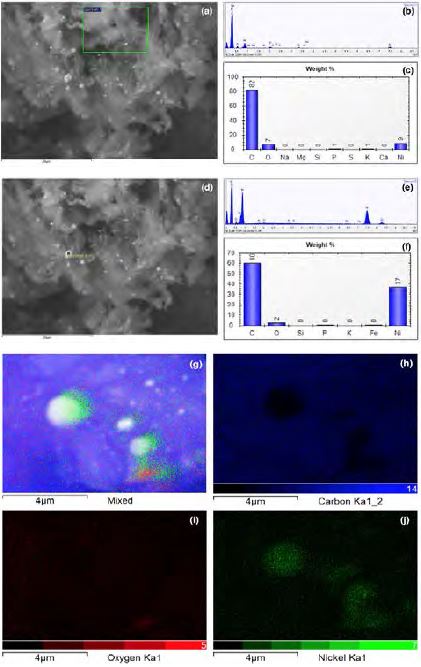
(a and d) Selected area for EDX analysis (b and c) EDX spectra of wide area of NiNPs/Carbon, (e and f) EDX spectra of the specific area (shown in a and d) of NiNPs/Carbon, (g-j) EDX mapping of NiNPs/Carbon.
The crystal structure of the NiNPs/Carbon particles was investigated by XRD. The XRD pattern of NiNPs/Carbon is shown in Figure 3. Four peaks were observed at diffraction angles of 25.88°, 44.35°, 51.70°, and 76.25° corresponding to the C (002), Ni (111), Ni (200), and Ni (220) planes, respectively. The XRD pattern confirmed that the NiNPs obtained by the solution plasma method showed high crystallinity and had an fcc structure [13][14]. The C (002) peak was broad, indicating the amorphous nature of the carbon particles. Moreover, the intensity of the NiNP diffraction peaks was very high, which indicates the high yield of NiNPs synthesized by the solution plasma method. The crystallite size of NiNPs (Ni (111) diffraction plane) was found to be 64 nm, as calculated by the Scherrer equation [13]-[15].
3.2 Crystal structure observation by XRD
The crystal structure of the NiNPs/Carbon particles was investigated by XRD. The XRD pattern of NiNPs/Carbon is shown in Figure 3. Four peaks were observed at diffraction angles of 25.88°, 44.35°, 51.70°, and 76.25° corresponding to the C (002), Ni (111), Ni (200), and Ni (220) planes, respectively. The XRD pattern confirmed that the NiNPs obtained by the solution plasma method showed high crystallinity and had an fcc structure [13][14]. The C (002) peak was broad, indicating the amorphous nature of the carbon particles. Moreover, the intensity of the NiNP diffraction peaks was very high, which indicates the high yield of NiNPs synthesized by the solution plasma method. The crystallite size of NiNPs (Ni (111) diffraction plane) was found to be 64 nm, as calculated by the Scherrer equation [13]-[15].
3.3 Catalytic activity analysis by CV
The CV test was performed in the voltage range of -0.2 – 1.4 V at a scan rate of 50 mV/s in a 0.1 M H2SO4 solution under O2-saturated conditions. The cyclic voltammogram shown in Figure 4 indicates that NiNPs/Carbon did not exhibit a significant electrocatalytic activity towards both the oxidation and reduction reactions. These results are not consistent with those reported by Sanli and Aytac. They claimed that Ni/Carbon shows catalytic activity towards oxygen reduction in acid solutions (0.1 M H2SO4) [16]. The CV results obtained by us suggest that NiNPs/Carbon is not catalytically active in an acidic medium.
4. Conclusions
The NiNPs-embedded carbon particles were successfully synthesized by solution plasma processing using waste vegetable oil as the carbon precursor. NiNPs were formed by the sputtering of the Ni electrodes and the carbon particles were obtained simultaneously from the waste vegetable oil during the solution plasma discharge. The SEM and XRD results revealed that the spherical NiNPs were embedded in the carbon-particle matrix and had a crystallite size of about 64 nm. Although the CV results indicated that NiNPs/Carbon could not catalyze oxygen reduction in acidic media, it is expected to be a promising catalyst for other processes such as the CO conversion, cyclization, and cycloaddition of organic compounds. The synthesis method proposed here is not only simple but also utilizes waste oil, which makes it economic. The NiNPs/Carbon produced in this study is expected to be a promising catalyst. The solution plasma method used in this study can also be used to synthesize other related materials.
Acknowledgments
This paper is extended and updated from the short version that appeared in the Proceedings of the International Symposium on Marine Engineering and Technology (ISMT 2016), held at Korea Maritime and Ocean University, Busan, Korea, November 3–4, 2016.
References
-
O. Takai, “Solution plasma processing (SPP),”, Pure and Applied Chemistry, 80(9), p2003-2011, (2008).
[https://doi.org/10.1351/pac200880092003]
-
P. Pootawang, N. Saito, O. Takai, and S. Y. Lee, “Rapid synthesis of ordered hexagonal mesoporous silica and their incorporation with Ag nanoparticles by solution plasma,”, Materials Research Bulletin, 47(10), p2726-2729, (2012).
[https://doi.org/10.1016/j.materresbull.2012.04.040]
-
P. Pootawang, N. Saito, and O. Takai, “Solution plasma process for template removal in mesoporous silica synthesis,”, Japanese Journal of Applied Physics, 49(12R), pp. 126202-1-7, (2010).
[https://doi.org/10.1143/JJAP.49.126202]
-
P. Pootawang, N. Saito, O. Takai, and S. Y. Lee, “Synthesis and characteristics of Ag/Pt bimetallic nanocomposites by arc-discharge solution plasma processing,”, Nanotechnology, 23, pp. 395602-1-8, (2012).
[https://doi.org/10.1088/0957-4484/23/39/395602]
-
P. Pootawang, N. Saito, and S. Y. Lee, “Discharge time dependence of a solution plasma process for colloidal copper nanoparticle synthesis and particle characteristics,”, Nanotechnology, 24, pp. 055604-1-9, (2013).
[https://doi.org/10.1088/0957-4484/24/5/055604]
-
J. Kang, O. L. Li, and N. Saito, “A simple synthesis method for nano-metal catalyst supported on mesoporous carbon: the solution plasma process,”, Nanoscale, 5, p6874-6882, (2013).
[https://doi.org/10.1039/c3nr01229h]
-
J. Kang, and N. Saito, “In situ solution plasma synthesis of mesoporous nanocarbon-supported bimetallic nanoparticles,”, RSC Advances, 5, p29131-29134, (2015).
[https://doi.org/10.1039/C5RA04220H]
-
D. W. Kim, O. L. Li, P. Pootawang, and N. Saito, “Solution plasma synthesis process of tungsten carbide on N-doped carbon nanocomposite with enhanced catalytic ORR activity and durability,”, RSC Advances, 4, p16813-16819, (2014).
[https://doi.org/10.1039/c4ra02380c]
-
Y. G. Morozov, O. V. Belousova, and M. V. Kuznetsov, “Preparation of nickel nanoparticles for catalytic applications,”, Inorganic Materials, 47(1), p36-40, (2011).
[https://doi.org/10.1134/S0020168510121027]
-
V. P. Ananikov, “Nickel: the “spirited horse” of transition metal catalysis,”, ACS Catalysis, 5, p1964-1971, (2015).
[https://doi.org/10.1021/acscatal.5b00072]
-
B. Li, S. Amiruddin, and J. Prakash, “A kinetics study of oxygen reduction reaction on Palladium-Nickel alloy,”, ECS Transactions, 11(1), p235-240, (2007).
[https://doi.org/10.1149/1.2780935]
- G. Pansuwan, S. Phuksawattanachai, K. Kerdthip, N. Sungworawongpana, S. Nounjeen, S. Anantachaisilp, J. Kang, G. Panomsuwan, T. Ueno, N. Saito, and P. Pootawang, “Nickel Nanoparticles-embedded Carbon particle fabrication by solution plasma in waste vegetable oil,”, Proceedings of the ISMT 2016, p68, (2016).
-
R. A. Landa, P. S. Antonel, M. M. Ruiz, O. E. Perez, A. Butera, G. Jorge, C. L. P. Oliveira, and R. M. Negri, “Magnetic and elastic anisotropy in magnetorheological elastomers using nickel-based nanoparticles and nanochains,”, Journal of Applied Physics, 114, pp. 213912-1-11, (2013).
[https://doi.org/10.1063/1.4839735]
-
J. Geng, D. A. Jefferson, and B. F. G. Johnson, “The unusual nanostructure of nickel-boron catalyst,”, Chemical Communications, p969-971, (2007).
[https://doi.org/10.1039/B615529D]
-
Y. Hou, H. Kondoh, T. Ohta, and S. Gao, “Size-controlled synthesis of nickel nanoparticles,”, Applied Surface Science, 241, p218-222, (2005).
[https://doi.org/10.1016/j.apsusc.2004.09.045]
-
A. E. Sanli, and A. Aytac, “Electrochemistry of the nickel electrode as a cathode catalyst in the media of acidic peroxide for application of the peroxide fuel cell,”, ECS Transactions, 42(1), p3-22, (2012).
[https://doi.org/10.1149/1.4705474]