
Degradation of synthetic dye in water by solution plasma process

Copyright © The Korean Society of Marine Engineering
This is an Open Access article distributed under the terms of the Creative Commons Attribution Non-Commercial License (http://creativecommons.org/licenses/by-nc/3.0), which permits unrestricted non-commercial use, distribution, and reproduction in any medium, provided the original work is properly cited.
Abstract
In this study, the solution plasma process was utilized with the aim of degrading synthetic dyes in water at atmospheric pressure. The experiments were conducted in a batch-type reactor consisting of a symmetric wire-wire electrode configuration with rhodamine B (RhB) as the target synthetic dye. The effects of the plasma treatment time and initial dye concentration on the RhB degradation were investigated by monitoring the change in absorbance of RhB solutions. The RhB solutions turned lighter in color and finally colorless with prolonged plasma treatment time, indicating the destruction of dye molecules. The RhB solutions were found to have degraded, following the first-order kinetic process. However, for high initial RhB concentrations, another kinetic process or factor seems to play a dominant role at the initial degradation stage. The fitted first-order rate constant decreased as the initial concentration increased. This result suggests that the degradation behavior and kinetic process of the RhB solution strongly depends on its initial concentration. The RhB degradation is considered to be due to a combination of factors, including the formation of chemically oxidative species, as well as the emission of intense UV radiation and high-energy electrons from the plasma. We believe that the solution plasma process may prove to be an effective and environment-friendly method for the degradation or remediation of synthetic dye in wastewater.
Keywords:
Wasterwater treatment, Advanced oxidation process, Solution plasma, Synthetic dyes1. Introduction
Synthetic dyes have been used extensively in a variety of manufacturing industries such as the textile, paper, cosmetics, leather, and printing industries [1][2]. The wide variety of colors originating from them makes various products more beautiful and fashionable, as well as improves their design and function. However, owing to the major presence of a toxic aromatic ring in them, wastewater streams containing synthetic dyes from industrial processes have become a serious environmental problem and human health issue at a broad range of levels from the local to the global [2]-[4]. In general, industrial wastewaters are treated by one or a combination of chemical, physical, and biological methods before their release into the local environment [5]-[9]. However, these conventional methods are ineffective and may be not suitable for the complete degradation of synthetic dye in wastewater streams. Therefore, the development of a universal effective method with environmental compatibility for the degradation or remediation of synthetic dyes is highly important in industrial dye wastewater treatment.
Recently, non-thermal plasma in liquid has emerged as an environment-friendly and effective technological method for the degradation of various synthetic dyes and toxic compounds [10]-[15]. In general, the non-thermal plasma is produced by applying high-voltage pulses with fast rise times and short durations at ambient conditions. When the plasma occurs in water, it initiates several physical and chemical processes, such as ultraviolet (UV) radiation, shock waves, high-energy electrons, and the formation of various chemically active species [15][16]. The hydroxyl radical and atomic oxygen are known be important reactive species, which play a major role in the degradation of organic pollutants in wastewater through oxidation reactions because of their high oxidation potentials [17]-[19]. Although several studies have been conducted on non-thermal plasma through different batch-type reactors and electrode geometries, its application to wastewater treatment has only very recently been studied, and there is a great necessity for further intense studies on various types of dyes.
In this study, non-thermal plasma generated in liquid water, termed “solution plasma,” was utilized for the degradation of synthetic dye at atmospheric pressure. A batch-type reactor consisting of a symmetric wire-wire electrode configuration was employed for the generation of the plasma. Rhodamine B, a member of the xanthenes dye family, was selected as the target synthetic dye. The effects of plasma treatment time and initial dye concentration on the degradation of RhB dyes were studied and discussed.
2. Experimental
2.1Materials
Rhodamine B (RhB, C28H31ClN2O3) dye was purchased from Sigma-Aldrich. Potassium chloride (KCl, 99.9% purity) was purchased from Ajax Finechem. Tungsten wire (99.9% purity) with a diameter of 1 mm was purchased from The Nilaco Corporation. Deionized water was used throughout the experiments in this study. All chemicals were of analytical grade and were used as received without further purification.
2.2Degradation of RhB by Solution Plasma
The synthetic wastewaters were prepared by dissolving the RhB dye with the deionized water at four initial concentrations (1, 5, 10, and 15 mg L−1). A specific amount of KCl was then added to the RhB solutions to increase the solution conductivity and stabilize the plasma during treatment. The prepared RhB solutions were magnetically stirred in a dark environment at room temperature for 24 h to reach the equilibrium condition. After this process, a quantity of 170 mL of each RhB solution was poured into a batchtype reactor made of acrylic glass with dimensions of 7.5 cm × 7.5 cm × 5 cm. A symmetric pair of 1-mm diameter tungsten electrodes shielded with insulating ceramic tubes was placed at the center of this reactor with a gap distance of approximately 0.9 mm. The prepared RhB solution served as a dielectric barrier between the two electrodes in the reactor. A schematic diagram of the experimental setup for the RhB degradation is shown in Figure 1. A bipolar pulsed voltage was applied to the tungsten electrodes using an MPP-HV02 Pekuris power generator (Kurita Seisakusho Co., Ltd.). The pulse duration and repetition frequency were fixed at 1.5 μs and 30 kHz, respectively. The plasma treatment times for the RhB solutions of differing initial concentrations was different, and depended on the time required for the RhB solution to turn colorless. The experiment was performed three times for each RhB solution of differing initial concentration to confirm its repeatability. The waveforms of discharge voltage and current were measured using a Yokogawa 701926 high-voltage probe and a Yokogawa 701929 current probe, respectively, connected to a Yokogawa DLM2024 oscilloscope. The discharge voltage and current were determined to be approximately 450 V and 6 A, respectively (Figure 2).
2.3Characterizations
The RhB degradation was monitored by measuring the change in the maximum absorbance at 554 nm using a Shimadzu UV-1800 UV-Vis spectrophotometer at room temperature. To measure the absorbance, a 2 μL quantity of RhB solution was collected from the reactor every 30 min during the treatment without stopping the reaction. The absorption spectra were recorded in the wavelength range of 300 to 700 nm with a quartz cell (1 cm path length).
To evaluate the formation of reactive species, the optical emission spectrum of the plasma was recorded with an Avantes AvaSpec-3648 optical spectrometer in the wavelength range of 200 to 900 nm. The spectrum was recorded by averaging three scans with a 200 ms integration time.
3. Results and Discussion
For each RhB solution of differing initial concentration, the absorption spectra after various durations of the plasma treatment are displayed in Figure 3. It was observed that the absorption peaks at 554 nm showed similar trends for all of the RhB solutions, with a continuous decrease in absorbance for an increase in treatment time. The corresponding decolorization of each RhB solution is shown in the inset photographs. As can be clearly seen, the color of each RhB solution gradually changed from pink to light pink, and finally to colorless, which is consistent with the decrease in the absorption peak at 554 nm. Additionally, a gradual decrease in the absorption peak at the wavelength of 354 nm was also observed. The decrease in the absorption peaks at 354 and 554 nm clearly indicates the destruction of the RhB dye molecules after plasma treatment. The degradation efficiency for each RhB solution of differing initial concentration can be calculated using Equation (1).
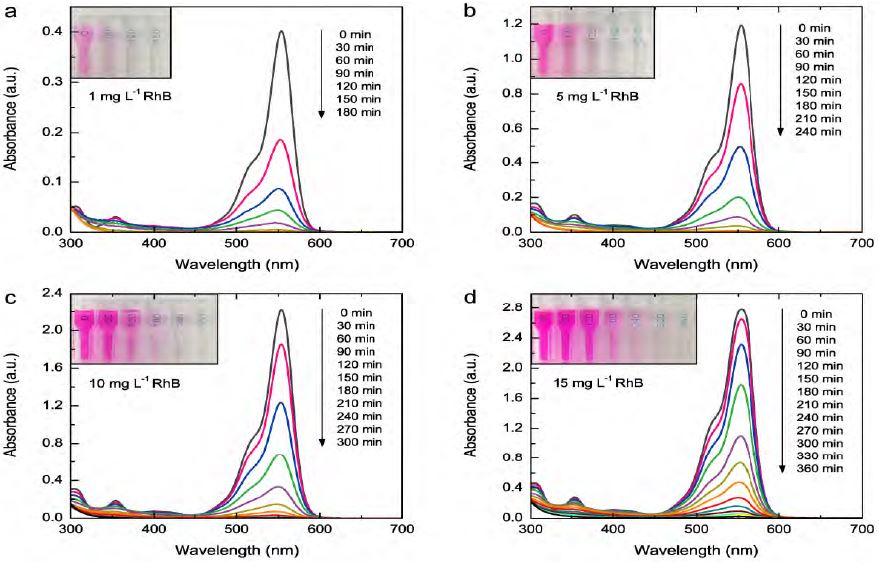
UV-Vis absorption spectra for RhB solutions of differing initial concentrations. For each RhB solution, the absorption spectra after various durations of plasma treatment are shown. The inset photograph for each RhB solution shows the corresponding decolorization after various treatment times.
(1) |
where C0 is the initial concentration of the RhB solution, and Ct is the residual concentration of the RhB solution after plasma treatment at time t. The RhB concentration was determined from the absorbance at 554 nm according to the Beer-Lambert law. Figure 4 a shows a plot of the degradation efficiency of the RhB solutions of differing initial concentrations as a function of the treatment time. The degradation efficiency of the RhB solution with an initial concentration of 1 mg L−1 RhB reached almost 100% within 180 min, while that for RhB solutions with higher initial concentrations required longer treatment times to reach 100%. The complete degradation of the RhB solutions with initial concentrations of 5, 10, and 15 mg L−1 was observed after a treatment time of approximately 210, 270, and 330 min, respectively. This result clearly indicates that the rate of increase of degradation efficiency decreased significantly when the initial concentration of the RhB solution was increased from 1 to 15 mg L−1. To evaluate the effect of the initial concentration on degradation kinetics further, where the degradation rate depends on the amount of dye molecules in the solution, the first-order rate constant of RhB degradation can be calculated using Equation (2):
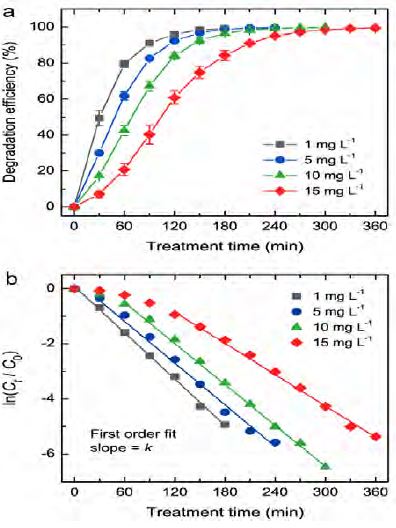
(a) Degradation efficiency as a function of treatment time for RhB solutions of differing initial concentrations. The vertical error bars were determined based on the standard deviation of three repeated experiments. (b) Plot of ln(Ct/C0) as a function of treatment time for RhB solutions of differing initial concentrations.
(2) |
where k is the first-order rate constant (min−1). Figure 4 (b) shows the plot of ln(Ct/C0) as a function of treatment time for Figure 4: (a) Degradation efficiency as a function of treatment time for RhB solutions of differing initial concentrations. The vertical error bars were determined based on the standard deviation of three repeated experiments. (b) Plot of ln(Ct/C0) as a function of treatment time for RhB solutions of differing initial concentrations. RhB initial concentrations according to Equation (2). The first-order rate constant for the degradation of each RhB solution was estimated from the slope of the straight line that most closely matched the experimental data. It was found that the degradation of the RhB solution with an initial concentration of 1 mg L-1 revealed a good linearity throughout the treatment time investigated, indicating its entire degradation via the first-order kinetic process. However, for the RhB solution with an initial concentration of 5 mg L-1, the experimental data slightly deviated from the linear fitting curve at the initial stage of treatment. This deviation became more obvious for the RhB solutions with initial concentrations of 10 and 15 mg L-1. The degradation via the first-order kinetic process began to occur after treatment times of 30, 60, and 120 min for the RhB solutions with initial concentrations of 5, 10, and 15 mg L-1, respectively. The first-order rate constants were calculated to be 0.028, 0.026, 0.025, and 0.019 min-1 for the RhB solutions with initial concentrations of 1, 5, 10, 15 mg L-1, respectively. These results are in agreement with previous findings that the rate constant decreases with an increase in the dye concentration [20]. From the results described above, three main points were confirmed, as follows. 1) The initial concentration of the RhB solution had a significant influence on the RhB degradation, and the degradation rate decreased with an increase in the initial concentration. 2) The RhB degradation entirely followed the first-order kinetic process when the RhB solutions had sufficiently low initial concentrations. 3) At higher concentrations, another kinetic process or factor seemed to play a dominant role in the degradation process. However, this kinetic process or factor could not be determined. Therefore, future studies need to clarify the process or factor at play.
To gain a better understanding of the RhB degradation by solution plasma, optical emission spectroscopic measurements were carried out to investigate the chemically reactive species formed in the plasma during treatment. Figure 5 shows the optical emission spectrum of the plasma generated in water in the wavelength range of 200 to 900 nm. The emission peaks at 434, 486, and 656 could be detected, corresponding to atomic Hγ, Hβ and Hα respectively. At higher wavelengths, the emission peaks attributed to atomic O were clearly observed at 778 and 844 nm. Additionally, a weak peak corresponding to the OH radical was also detectable at the shorter wavelength of 310 nm [21]-[23]. It is considered that the OH radical and atomic O generated from the plasma play important roles in initiating the degradation of the RhB dye molecules in the solution owing to their strong oxidative potentials. The oxidative species can diffuse into the gas phase and dissolve in water through the interface layer to react with the RhB dye in water resulting in degradation into its derivative, as reflected by the decolorization of RhB solution. In addition to the chemically oxidative species, the simultaneous occurrence of intense UV radiation and high-energy electrons from the plasma may also have a synergistic contribution to the degradation of the RhB solution. The UV light can enhance the water dissociation reaction and subsequently increase the formation of OH radicals. The more energetic electrons produced under the strong electric field between the electrodes may also help to dissociate the dye molecules.
4. Conclusions
The degradation of RhB solutions of differing initial concentrations was investigated using a solution plasma process at atmospheric pressure. The results showed that the RhB solutions were degraded continuously with an increase in the treatment time, as indicated by the decolorization of the solutions. For RhB solutions of higher initial concentrations, a longer treatment time was required for complete degradation. As the initial concentration increased, longer treatment times were required before the RhB degradation began to proceed following the first-order kinetic process. For the solutions with higher initial concentrations, another kinetic process or factor may play a role in the degradation at the initial degradation stage. The formation of chemically oxidative species (i.e., OH radicals and atomic O) are believed to play major roles in the degradation of the RhB solution, while the intense UV radiation and high-energy electrons seem to have minor contributions. The results obtained in this study show that the solution plasma process has the potential to become a useful method for the degradation or remediation of synthetic dyes in wastewaters because of its capability of effective degradation as well as its environmental compatibility. We believe that this process can be further developed to large-scale and continuous process applications of dye wastewater treatment using multiple plasma channels with a continuous flow system.
Acknowledgments
The authors gratefully thank the financial support from Nagoya University for the joint research projects between Chulalongkorn University and Nagoya University via the research grant for the NU-PPC Plasma Chemical Technology Laboratory at Chulalongkorn University, Thailand.
This paper is extended and updated from the short version that appeared in the Proceedings of the International Symposium on Marine Engineering and Technology (ISMT 2016), held at Korea Maritime and Ocean University, Busan, Korea, November 3–4, 2016.
References
- M. Clark, Handbook of Textile and Industrial Dyeing, Volume 1: Principles, Processes and Types of Dyes, 1st Ed., Woodhead Publishing, (2011).
- V. M. Correia, T. Stephenson, and S. J. Judd, Characterization of textile wastewater−A Review, 15(10), p917-929, (1994).
- R. M. Christie, Environmental Aspects of Textile Dyeing, 1st Ed., Woodhead Publishing, (2007).
- A. Reife, and H. S. Freeman, Environmental Chemistry of Dyes and Pigments, 1st Ed., John Wiley & Sons, Inc., (1996).
-
P. C. Vandevivere, R. Bianchi, and W. Verstrate, “Treatment and reuse of wastewater from the textile wetprocessing industry: Review of emerging technologies,”, Journal of Chemical Technology and Biotechnology, 72(4), p289-302, (1998).
[https://doi.org/10.1002/(SICI)1097-4660(199808)72:4<289::AID-JCTB905>3.0.CO;2-#]
-
V. K. Gupta, and Suhas, “Application of low-cost adsorbents for dye removal–A review,”, Journal of Environmental Management, 90(8), p2313-2342, (2009).
[https://doi.org/10.1016/j.jenvman.2008.11.017]
-
H. An, Y. Qian, X. Gu, and W. Z. Tang, “Biological treatment of dye wastewaters using an anaerobic-oxic system,”, Chemosphere, 33(12), p2533-2542, (1996).
[https://doi.org/10.1016/S0045-6535(96)00349-9]
-
S. Wang, H. Li, S. Xie, S. Liu, and L. Xu, “Physical and chemical regeneration of zeolitic adsorbents for dye removal in wastewater treatment,”, Chemosphere, 65(1), p82-87, (2006).
[https://doi.org/10.1016/j.chemosphere.2006.02.043]
-
C. S. D. Rodrigues, L. M. Madeira, and R. A. R. Boaventura, “Decontamination of an industrial cotton dyeing wastewater by chemical and biological processes,”, Industrial & Engineering Chemistry Research, 53(6), p2412-2421, (2014).
[https://doi.org/10.1021/ie402750p]
-
B. Sun, M. Sato, and J. S. Clements, “Use of a pulsed high-voltage discharge for removal of organic compounds in aqueous solution,”, Journal of Physics D: Applied Physics, 32(15), p1908, (1999).
[https://doi.org/10.1088/0022-3727/32/15/319]
-
M. A. Malik, A. Ghaffar, and S. A. Malik, Water purification by electrical discharge,”, Plasma Sources Science and Technology, 10(1), p82-91, (2001).
[https://doi.org/10.1088/0963-0252/10/1/311]
-
P. Bruggeman, and C. Leys, “Non-thermal plasmas in and in contact with liquids,”, Journal of Physics D: Applied Physics, 42(5), p053001, (2009).
[https://doi.org/10.1088/0022-3727/42/5/053001]
-
G. An, Y. Sun, T. Zhu, and X. Yan, “Degradation of phenol in mists by a non-thermal plasma reactor,”, Chemosphere, 84(9), p1296-1300, (2011).
[https://doi.org/10.1016/j.chemosphere.2011.05.007]
-
M. Tezuka, and M. Iwasaki, “Plasma induced degradation of chlorophenols in an aqueous solution,”, Thin Solid Films, 316(1-2), p123-127, (1998).
[https://doi.org/10.1016/S0040-6090(98)00401-5]
-
B. Jiang, J. Zheng, Q. Liu, and M. Wu, “Review on electrical discharge plasma technology for wastewater remediation,”, Chemical Engineering Journal, 236, p348-368, (2014).
[https://doi.org/10.1016/j.cej.2013.09.090]
-
P. Sunka, V. Babicky, M. Clupek, P. Lukes, M. Simek, J. Schmidt, and M. Cernák, “Generation of chemically active species by electrical discharges in water,”, Plasma Sources Science and Technology, 8(2), p258-265, (1999).
[https://doi.org/10.1088/0963-0252/8/2/006]
-
J. L. Wang, and L. J. Xu, “Advanced oxidation processes for wastewater treatment: Formation of hydroxyl radical and application,”, Critical Reviews in Environmental Science and Technology, 42(3), p251-325, (2011).
[https://doi.org/10.1080/10643389.2010.507698]
-
S. S. Hinde, C. H. Bhosale, K. Y. Rajpure, and J. H. Lee, “Remediation of wastewater: Role of hydroxyl radicals,”, Journal of Photochemistry and Photobiology B: Biology, 141, p210-216, (2014).
[https://doi.org/10.1016/j.jphotobiol.2014.10.015]
- G. Panomsuwan, T. Morishita, J. Kang, R. Rujiravanit, T. Ueno, and N. Saito, “Degradation of synthetic dye in water by solution plasma process,”, Proceedings of the International Symposium on Marine Engineering and Technology (ISMT 2016), p67, (2016).
- D. Piroi, M. Magureanu, N. B. Mandache, and V. I. Parvulescu, “Decomposition of organic dyes in water using non-thermal plasma,”, Proceeding of 19th International Symposium on Plasma Chemistry, (2009).
-
P. Bruggeman, T Verreycken, M. A. Gonzalez, J. L. Walsh, M. G. Kong, C. Leys, D. C. Schram, “Optical emission spectroscopy as a diagnostic for plasmas in liquids: Opportunities and pitfalls,”, Journal of Physics D: Applied Physics, 43(12), p124005, (2010).
[https://doi.org/10.1088/0022-3727/43/12/124005]
-
C. Miron, M. A. Bratescu, N. Saito, O. Takai, “Timeresolved optical emission spectroscopy in water electrical discharges,”, Plasma Chemistry and Plasma Processing, 30(5), p619-631, (2010).
[https://doi.org/10.1007/s11090-010-9248-1]
-
B. Sun, M. Sato, J. S. Clemens, “Optical study of active species produced by a pulsed streamer corona discharge in water,”, Journal of Electrostatics, 39(3), p189-202, (1997).
[https://doi.org/10.1016/S0304-3886(97)00002-8]