
Microstructural behavior on weld fusion zone of Al-Ti and Ti-Al dissimilar lap welding using single-mode fiber laser
Titanium (Ti) metal and its alloys are desirable materials for ship hulls and other structures because of their high strength, light weight and corrosion-resistance. And light weight and corrosion-resistant aluminum (Al) is the ideal metal for shipbuilding. The joining of Ti and Al dissimilar metals is one of the effective measures to reduce weight of the structures or to save rare metals. Ti and Al have great differences in materials properties, and intermetallic compounds such as Ti3Al, TiAl, TiAl3 are easily formed at the contacting surface between Ti and Al. Thus, welding or joining of Ti and Al is considered to be extremely difficult. However, it was clarified that ultra-high speed welding could suppress the formation of intermetallic compounds in the previous study. Results of tensile shear strength increases with an increase in the welding speed, and therefore extremely high welding speed (50m/min in this study) is good to dissimilar weldability for Ti and Al. In this study, therefore, full penetration dissimilar lap welding of Ti (upper) - Al (lower) and Al (upper) - Ti (lower) with single-mode fiber laser was tried at ultra-high welding speed, and the microstructure of the interface zones in the dissimilar Al and Ti weld beads was investigated.
Keywords:
Ti, Al, Dissimilar welding, Laser, Intermetallic compound1. Introduction
Dissimilar welding has been received great attention because of good advantages such as reducing weight, improving material properties, supplementing each material and saving rare metals. Ti metal and its alloys are desirable materials for ship hulls and other structures because of their high strength, light weight and corrosion-resistance. And light weight and corrosion-resistant Al is the ideal metal for shipbuilding. If constructed in Ti and Al, ships would have lighter weight for the same size-allowing for a bigger payload-and virtually no corrosion. But because Ti costs up to nine times more than steel, and Al and Ti are technically difficult and expensive to manufacture into marine vessel hulls, it has been avoided by the shipbuilding industry. As an implication there is need for a method to utilize the superior properties of dissimilar materials such as corrosive properties and strength of Ti and low weight and low cost of Al. Several approaches to weld Ti to Al have been made in the past. In the previous researches, to reduce generation of intermetallic compounds, the filler metal, and FSW welding and typical welding arrangement butt joint were used to control material percentage due to weld location. Also, butt joint was usually performed to weld the Ti and Al dissimilar metals [1]-[5] but there were few reports of lap welding of these alloys. Therefore, a study of the lap welding process of Ti and Al compound structures is important. The difference in combination of Ti and Al or Al and Ti lap sheets was investigated as an important viewpoint in dissimilar welding in this study.
2. Materials and Experimental Procedures
2.1 Materials Used
The materials used in experiments were pure titanium and commercially available aluminum A1050 sheets of 0.3 mm thickness, 30 mm width and 60 mm length. The Titanium has over 99.9% Ti and Al050 has about 99.57% Al. Physical properties of materials used show Table 1. The performances of Ti and Al have great differences in lattice structure, melting point, heat conductivity, coefficient of linear expansion, etc. During the welding process, Al is lost at the temperature below the melting point of Ti. Composition of the weld metal is asymmetric. In addition, the laminated Ti and Al intermetallic com pounds such as Ti3Al, TiAl, TiAl3, etc., are easily formed near the contacting surface between Ti and Al as shown Figure 1. As a result, it is difficult to weld the Ti and Al materials.
2.2 Experimental Procedures
A single-mode fiber laser has several magnificent merits in comparison with the others heat sources of welding. It has high quality and high efficiency, and it can be easily focused to an extremely small spot than others. And thereby an ultra-high power density can be achieved. Figure 2 shows comparison of a power density among typical lasers. Furthermore, a single-mode fiber laser is compact and has a long life.
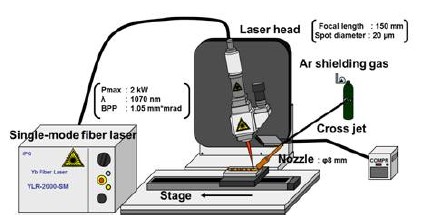
Schematic experimental set-up for 2 kW single-mode fiber laser welding of Al and Ti dissimilar metals.
The laser with a higher power/energy density can produce a deeper penetration weld or achieve a higher welding speed than lasers with a lower power density at the same powers [6]. In this study, therefore, laser lap welding of Ti and Al dissimilar sheets was performed with a single-mode fiber laser at an extremely high welding speed. Microstructural behavior and formation phases of the weld fusion zones in Ti and Al were investigated.
Figure 3 shows the experimental device installed for dissimilar welding of Ti and Al. 0.3mm thick Ti and Al workpieces were fixed to a jig on high speed stage. The single-mode fiber laser with the maximum power of 2 kW and the wavelength of 1070 nm was utilized, and a laser beam parameter product (BPP) was 1.05 mm∙mrad. The laser beam was delivered by an optical fiber and focused on the specimen surface by lens of 150mm in focal length. The spot size of a laser beam was about 20 ㎛ at the focal point. A laser beam was directly irradiated on 0.3 mm thick material sheets overlapped. Dissimilar welding conditions used in the experiments were the laser power of 1 kW, the welding speed of 5, 10, 20, 30, 40 and 50 m/min and the defocused distance of 0 mm. Ar shielding gas of 35 L/min was used to suppress oxidation of the upper molten surface during lap welding.
The strengths of the dissimilar welding were evaluated by the tensile shear test at the traveling speed of 0.1 mm/s. Microstructure of the cross sections of the weld parts was observed by microscope (SEM), energy dispersive X-ray spectroscopy (EDX) and transmission electron microscopy (TEM) to investigate the weld fusion zones for the production of a strong weld resulting from the high-speed welding process.
3. Results and Discussion
3.1 Ti and Al dissimilar welds produced with 16 kW disk laser at various high welding speeds
It is generally recognized until now that laser welding of Ti and Al dissimilar metals is too difficult to produce sound welds due to their formation of intermetallic compounds. In this study, full penetration lap welding of Ti and Al sheets was performed using disk laser under various conditions which had relatively good weld condition. Figure 4 shows the welds bead of Al-Ti and Ti-Al dissimilar welds made with disk laser. A laser power 1.25 kW and 9 m/min welding speed had best weldability of Al (upper) –Ti (lower) and 1kW laser power and 10m/min welding speed was good condition for Ti (upper) –Al (lower) in this study. But welds beads had cracks due to brittle intermetallic compounds under all conditions. Crack was generated easily in Al- Ti welds zone compare with Ti-Al conditions.
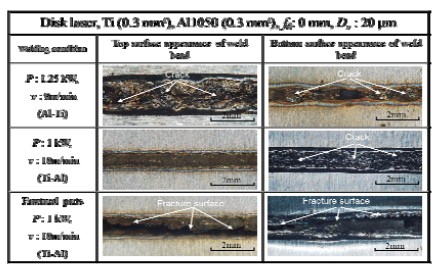
Photographs of top and bottom surface appearances and fractural parts photos of Ti and Al dissimilar welds made with disk laser at different welding conditions.
The mechanical properties of the welds were evaluated by the tensile shear test. The obtained loads of the tensile shear test for Al-Ti and Ti-Al were 565 N and 587N respectively. It was difficult to make sound welds because a spot size of laser beam was large compare with a thickness of workpiece, and a thickness of specimens was too thin and Al has very low viscosity to hold melt parts sufficiently.
3.2 Ti and Al dissimilar welds produced with single-mode fiber laser at various high welding speeds
In this study, lap welding of Ti and Al sheets was performed at extremely high welding speeds using a single-mode fiber laser to produce sound welds by the formation suppression of intermetallic compounds. Figure 5 shows top and bottom surface appearances of weld beads of Ti and Al dissimilar lap welds made with a single-mode fiber laser at 1kW power and various welding speeds from 10 to 50 m/min under the focal conditions. Under all the conditions, full penetration welds were obtained. Cracks were present in the weld bead of Ti side made at 10 m/min for the Al (upper)-Ti (lower) combination, but sound welds were formed in all the weld beads in Figure 5. The bead widths were getting narrower with increasing the welding speed. The mechanical properties of the welds were evaluated by the tensile shear test.
Figure 6 indicates the results of the tensile shear test. The longitudinal axes show the obtained loads of the tensile shear test. The tensile shear loads of Ti-Ti welds were the highest, while those of Al-Al welds were the smallest. The loads of the Al-Ti and Ti-Al dissimilar welds were slightly higher than those of Al-Al welds.
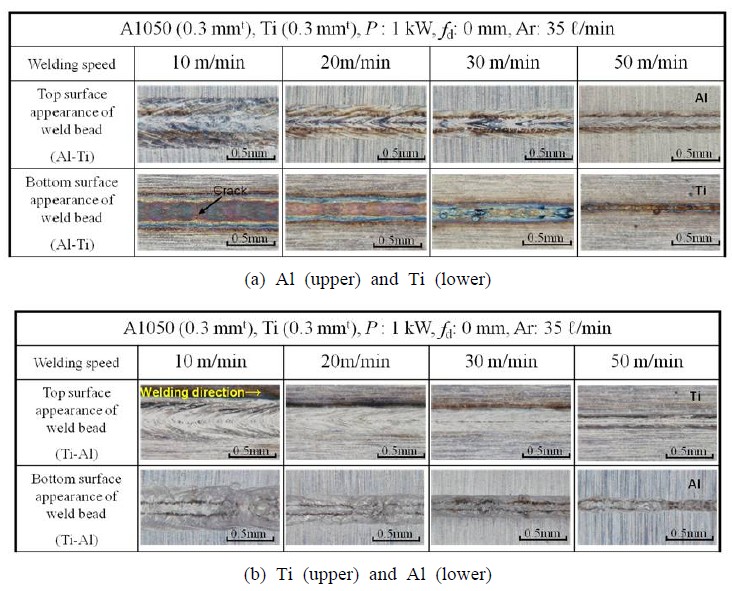
Photographs of top and bottom surface appearances of Ti and Al dissimilar welds made with single-mode laser at different welding conditions.
The loads of welds were about 700 N (for 20 mm wide) except Ti-Al welds produced at 40 and 50 m/min welding speed. It had higher tensile strength than results of disk laser welds which discussed in chapter 3.1. In the case of Ti-Ti and Al-Al similar welds, the fracture occurred in the weld beads, and accordingly the loads decreased with an increase in the welding speed due to the decreased welding area. In the most cases of Al-Ti and Ti-Al welds the fracture of the specimens occurred in the base Al, as shown in the polished cross sections of Figure 6 and thus the loads were almost equal and this the load were similar with value of 0.3 mm Al tensile shear load. The tensile shear strength increased with an increase in the welding speed accompanying with a decrease in the welding area, and therefore extremely high welding speed is good for dissimilar weldability for Al and Ti. When Al was located on the upper side of Ti, better weldability could be obtained than that of the opposite Ti-Al combination. Workpieces of Al-Ti dissimilar welds at 50 m/min were fractured at base metal nearby weld zones even though the weld beads were very narrow, and the reason may be the reduction in brittle intermetallic compounds leading to a strong joint of Al and Ti. On the other hand, Ti-Al dissimilar welds at 50m/min fractured in the weld zone. The difference may be attributed to the formation location of the Ti-rich zone and intermetallic compounds to the lapped interface.
The tensile shear test in this study shows a different tendency from the previous research results [1]-[4], showing easy fracture in Ti and Al welds zones produced only at high speeds due to brittle intermetallic compounds near the interface of two sheets, but the fracture in Al base metal in the other welds. To understand the effects of welding speed and material upper-lower combination on weldability, the microstructural behavior should be elucidated by TEM.
3.3 Microstructural characteristics of interface zone
Ti was locally distributed into Al sheets, but relatively very small amount of Al was distributed into Ti sheet because small amounts of Al are easily included in Ti as Ti solid solution. The zone including intermetallic compounds was changed by welding speed. If the welding speed increased, the weld zone was reduced [7]. To know the reason for the tendency difference of tensile shear test results, microstructural characteristics were analyzed. In order to understand the difference in welds characteristics by the change in welding speed and material set-up were investigated in detail. The formation tendency of microstructural phases and intermetallic compounds in weld fusion zones were subjected to XRD analysis in the previous study [7]. At the slower speed of 10 m/min, Al3Ti intermetallic compounds were detected in addition to Al and Ti elements of basemetals, while no peaks from intermetallic compounds were found and peaks from Al and Ti phases were only identified at speed of 50 m/min. It is moreover confirmed from the SEM photo that a larger area of Ti enriched zone was flowed into the melted Al fusion zone.
From the above results, it is supposed that intermetallic compounds were formed at the high speed of 10 m/min, resulting in the formation of cracks. On the other hand, the formation tendency of brittle intermetallic compounds should be reduced at the extremely high. Accordingly, the above result leads to high production possibility of strong dissimilar weld joints in Al and Ti sheets with a single-mode fiber laser at ultra-high welding speed.
To confirm the exact microstructure of laser welds, microstructure phases were observed and analyzed with TEM with EDX analysis. Figure 7 (a) and (b) show TEM images of the boundary layer near the Al and Ti mixed zone of dissimilar Al-Ti and Ti-Al lap sheets subjected to laser welding at the speed of 50 m/min. The small red box areas were analyzed with EDX, and the data were summarized using atomic percentages of Al and Ti and phase diagram system in Figure 1. Figure 7 red box areas exhibited formation phases of Al, Al3Ti, AlTi, Ti, Ti+Al3Ti, Ti+Al2Ti, Al2Ti, and Al, respectively.
The weld fusion zone was solidified at extremely high solidification rates, and therefore the growth of such phases was suppressed at high welding speeds. And different types of intermetallic compounds were generated depends on specimen location. Especially, Ti area of Al-Ti looks lamellar microstructure and needle-shaped martensite. Needle-shaped martensitic Ti phase was generated on Ti rich side of a weld fusion zone, and dendritic phases of intermetallic compounds were formed from Ti phase in Figure 7 (a).On the other hands, martensitic Ti phase was not generated on Ti rich side of a weld fusion zone, and small size dendritic phases and island type phases of intermetallic compounds were formed from Ti phase in Figure 7 (b).
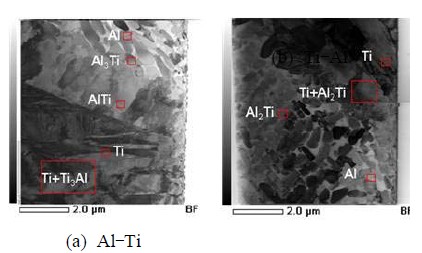
TEM photos and TEM results of laser weld fusion zones near lap joint interfaces of dissimilar Al (upper) – Ti (lower) and Ti (upper) – Al (lower) sheets produced at 50 m/min.
In this study, very small-sized grains and needle-shaped martensitic Ti phases were generated by extremely high melting and solidification rate during welding with a high-energy/power density laser beam. The amount of needle-shaped martensitic Ti phase generated under Al –Ti specimen set-up conditions at 50 m/min welding speed. This is in good agreement of the general interpretation of rapid solidification.
4. Conclusions
Ti and Al dissimilar lap welding was carried out at various welding speeds and Al-Ti and Ti-Al specimen combinations using single-mode fiber laser which had very high power density in order to find out the possibility of Ti and Al dissimilar lap welding. The results of the tensile shear test show that Al-Ti dissimilar weld joints had higher tensile shear load than Ti-Al. And single-mode laser welding process makes higher tensile shear load than disk laser dissimilar welding. The apparent tensile shear strength increased with increasing the welding speed under all conditions. According to microstructural observation, when the welding speed was 50 m/min high at 1 kW laser power, intermetallic compounds were sufficiently reduced. On the other hand, if the welding speed was 10 m/min low, intermetallic compounds were formed more easily than at high speed, but was enough to reduce brittle intermetallic compound formation using high energy density laser which had a very small spot size.
It was confirmed that the ultra-high welding speed of 50 m/min could reduce the formation area of brittle intermetallic compounds of Al3Ti and Al2Ti. And a needle-shaped martensitic Ti phase was generated under Al-Ti condition at 50m/min welding speed. On the other hands, martensitic Ti phase was not generated on Ti rich side of a weld fusion zone, and small size dendritic phases and island type phases of intermetallic compounds were formed from Ti phase under Ti-Al condition at 50 m/min welding speed.
Consequently, dissimilar lap welding of Al and Ti sheets using a single-mode fiber laser at ultra-high welding speeds suggests much production possibility of strong weld joints by reducing brittle intermetallic compounds. And specimen location is one of important factor of welding condition for dissimilar lap welding of Al and Ti sheets using a single-mode fiber laser at ultra-high welding speeds.
Acknowledgments
This work was conducted as a part of A-STEP “High-quality and high-efficiency processing technology of titanium alloy using a high brightness laser process control method”
This research was supported by the MSIP(Ministry of Science, ICT & Future Planning), Korea, under the ITRC (Information Technology Research Center) support program supervised by the NIPA (National IT Industry Promotion Agency (NIPA-2013-H0301-13-2009)
Notes
References
- C. Leyens, and M. Peters, Titanium and Titanium Alloys; Fundamentals and Applications, Weinheim, WILEY-VCH GmbH & Co. KGaA, (2003).
-
S. Chen, L. Li, Y. Chen, and J. Huang, “Joining mechanism of Ti/Al dissimilar alloys during laser welding-brazing process”, Journal of Alloys and Compounds, 509(3), p891-898, (2011).
[https://doi.org/10.1016/j.jallcom.2010.09.125]
-
Y. C. Chen, and K. Nakata, “Microstructural characterization and mechanical properties in friction stir welding of aluminum and titanium dissimilar alloys”, Materials and Design, 30(3), p469-474, (2009).
[https://doi.org/10.1016/j.matdes.2008.06.008]
- Katayama Seiji, “Laser welding”, Journal of the Japan welding society, 78(2), p124-138, (2009), (in Japanese).
- Katayama Seiji, “Laser welding for manufacturing innovation”, Journal of the Japan welding society, 78(8), p682-692, (2009), (in Japanese).
- Hideyasu Shamoto, and Kazuhisa Mikame, “The feature of high power single mode fiber laser processing”, Proceedings of the 72nd Laser Materials Processing Conference, p31-34, (2009).
-
S. J. Lee, H. Nakamura, Y. Kawahito, and S. Katayama, “Weldability of Ti and Al dissimilar metals using single-mode fiber laser”, Journal of Laser Micro/Nano engineering, 8(2), p149-154, (2013).
[https://doi.org/10.5781/KWJS.2013.31.5.64]