
A study on performance and smoke emission characteristics by blending low purity methanol in a DI diesel engine with the EGR rates of 0, 12.8 and 16.5%
The purpose of this study is to investigate experimentally the effect of low purity methanol (LPM) on performance and smoke emission characteristics by using a four-cycle, four-cylinder, water-cooled, direct injection diesel engine with EGR system. The experiments are performed by the change of engine load in the engine load ranges of 25 to 100% with an interval of 25% under the constant engine speed of 2000 rpm. The LPM in the fuel blends contained 24.88% water by volume. The blended fuel ratios of diesel oil to LPM are maintained at 100/0, 95/5, 90/10 and 85/15% on the volume basis. In this paper, EGR rates are varied in three conditions of 0, 12.8 and 16.5%. The result shows that the brake power of a blended fuel with 15% LPM is reduced more 11.1% than that of the neat diesel oil at the full load with the EGR rate of 16.5%. At this condition, also, the brake specific fuel consumption (BSFC) is increased by 3.2%, the exhaust gas temperature is decreased by 10.7%, the smoke opacity is decreased by 18.7% and the brake thermal efficiency is increased by 7.3%. The sharp reduction of smoke opacity for a blended fuel with the LPM content of 15% at the full load without EGR system is observed by 68.4% compared with that of the neat diesel oil due to the high oxygen content of LPM.
Keywords:
Low purity methanol (LPM), Smoke emission, Diesel engine, EGR rate, Brake power, Brake specific fuel consumption, Brake thermal efficiency, Exhaust gas temperature1. Introduction
The diesel engine in combustion devices is widely used for the transportation vehicle, agriculture support, power plant and factories due to the advantages of thermal efficiency, reliability and durability. However, diesel engines are formed more NOX and smoke emissions than gasoline engines. Therefore, the automotive manufacturers and researchers are attracted by the reduction countermeasures of NOX and smoke emissions in diesel engines[1][2].
NOX emissions could be controlled by the techniques such as EGR, SCR, etc.[3]-[7] The EGR (Exhaust gas recirculation) that recirculates exhaust gases into an intake manifold and mixes with fresh airs is a simple technique. The application of EGR results in the lower combustion temperature owing to the increase of specific heat and the decrease of oxygen concentration in the intake mixture[8]-[12]. This is the reduction reason of NOX emissions in diesel engines operating with EGR system.
Meanwhile, the application of EGR also affects on the smoke emission. The increase of EGR rate reduces the availability of oxygen for fuel combustion which causes the incomplete combustion resulting in the rise of smoke emission. Bae et al. [13]-[16] have conducted an experiment applying the EGR system with a scrubber system using water injection to eliminate smoke contents. They have shown smoke emission in exhaust gases is reduced by 20 to 70% when the scrubber system is applied in the range of experimental conditions. NOX emissions are markedly decreased, especially at higher loads, while smoke emission is increased owing to the decrease in intake and exhaust oxygen concentrations, and the increase in equivalence ratio as the EGR rate is elevated.
The concerns of automotive manufacturers and researchers not only reduce exhaust emissions due to the stringent regulation but also overcome the depletion of fossil fuel reserves. Therefore, as a method for solving these problems the study on renewable energy sources is taken into their consideration[17][18]. Zhu et al. [19] reported that NOX emissions decrease in proportion to the rise of alcohol (methanol and ethanol) in the blended fuel. They also found that the increase in rate of methanol in the fuel reduces smoke emission. Bae et al. [20] examined experimentally the characteristics of rice bran oil used as a fuel of diesel engine, and proved the oil is good enough to be used as the alternative fuel of diesel engine if the viscosity is improved. They reported that the brake specific fuel consumption and NOX emissions increase, while soot emission decreases as the blending rate of rice bran oil is enriched.
Another reduction effect of smoke emission among the exhaust emissions of diesel engine is the presence of H2O proportion inside the combustion chamber. H2O in the combustion of diesel engine may be obtained from the fresh air in a high humidity condition or recirculated exhaust gas for the diesel engine with EGR system as reported by Teini et al. [21] They investigated the effect of CO2/H2O on the formation of soot particles in the homogeneous environment of a rapid compression facility. They found that cyclopentapyrene (C18H10) formation, which is a representative compound to indicate large PAH formation and thus relative soot forming tendencies, reduces with increasing H2O percentage in the mixture.
Based on the study literatures above, the presences of alcohol and H2O in the combustion of diesel engine can reduce the smoke emission. The use of methanol as a fuel blend reduces the fossil fuel dependency. The objective of this study is to investigate the effect of low purity methanol, which contains the water content of 24.88% in the volume base, on performance characteristics and smoke emission in the direct injection (DI) diesel engine with EGR system.
2. Experimental Apparatus and Procedures
2.1 Experimental Apparatus
Figure 1 shows the schematic diagram of experimental system. Experiments were conducted on a naturally aspirated, water-cooled, four-cylinder, direct injection diesel engine. The specifications of the test engine are shown in Table 1.
A diesel engine ① is coupled with the hydraulic dynamometer ② in order to impose the variation of engine load which is supplied by dynamometer pump ③ from the water tank ④. The variations of engine load are set by adjusting the valve opening ⑥. Meanwhile, the EGR rate is changed by setting EGR valve opening ⑤. A heat exchanger ⑦ is set to adjust the temperature of recirculated exhaust gas. In the present paper, however, the heat exchanger is not applied. The U-tube manometers, ⑧ and ⑨, are used to measure the mass flow rates of recirculated exhaust gas and fresh air, respectively.
2.2 Experimental Procedures
The fuels used in this experiment adopted EURO II diesel oil produced by PERTAMINA (Indonesian Oil and Gas Company) and diesel-LPM blends in order to achieve the more effective reduction of exhaust emissions. The blended fuels contain 5, 10 and 15% by volume of LPM which are named as D95LPM5, D90LPM10 and D85LPM15, respectively. Properties of EURO II diesel oil and LPM are given in Table 2.
As shown in Table 2, the heating value of LPM is lower than that of EURO II diesel oil. The kinematic viscosity of LPM is very low compared with that of EURO II diesel oil. The cetane number of EURO II diesel oil is 48. Meanwhile, the LPM has the ce tane number of 3.3. The water content of LPM is close to 500 times higher than that of EURO II diesel oil.
At the constant engine speed of 2000 rpm, which is the point of maximum torque, the experiments were conducted in the engine load range of 25 to 100 % with an interval of 25%. The EGR rates of 0, 12.8 and 16.5% were set after the EGR rate was manually controlled by a mechanical throttle valve in the recirculation line. From engine load, EGR rate and blended fuel, two parameters were kept constant and only one parameter was varied. In this experiment, the variation of EGR rate is less than ± 2.8%, and the variation rate of engine load is less than ± 4%.
The exhaust gas temperatures, EGR gas inlet and outlet, and gases mixture (EGR gas and fresh air) were measured by using K-type thermocouples in which their values were monitored through the temperature displays (⑩ – ⑬). The cooling water temperature of engine was monitored by setting K-type thermocouple at the cooling water outlet and its value may be observed in ⑭. A tachometer ㉒ was set to measure the engine speed and its value may be monitored in ⑮. A load cell was imposed close to the dynamometer to measure the torque and could be monitored in ⑯. A fuel tank ⑰ was set on a burette ⑲ which was used to measure the fuel consumption rate. The fuel valves, ⑱ and ⑳, were set to regulate the fuel supply. The smoke emission emitted from diesel engine was measured by using a smoke meter ㉔.
3. Results and Discussions
3.1 Effect of LPM on Brake Specific Fuel Consumption
The BSFC is defined as the mass flow rate of fuel consumption to the brake power. In this experiment, the BSFC is decreased with increasing the engine load up to the engine load of 50% at a given EGR rate. The presence of LPM in the fuel blends increases the BSFC for almost all experimental conditions as shown in Figure 2 (a), (b) and (c). This is because the lower heating value of LPM-diesel blends is smaller than that of the neat diesel oil as well as brake power of Figure 3. This means that the fuel in the case of LPM-diesel blends must be more injected into the combustion chamber than the neat diesel oil in order to produce the same brake power.
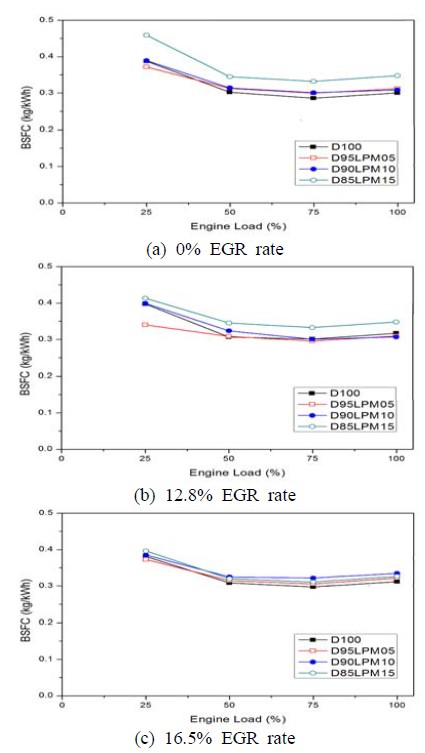
Effect of LPM proportion in the fuel blends on brake specific fuel consumption for respective engine load at a given EGR rate
The BSFC is increased to 18.4% when LPM content in the fuel blends rises up to 15% in comparing with that of the neat diesel oil for the case without EGR at the low engine load as seen in Figure 2 (a). In the case of full load, the BSFC is raised to 15.8% by increasing LPM to 15% in the fuel blends. The BSFC is decreased to 3.8% when the LPM is increased to 5% in the fuel blends compared with that of the neat diesel oil at the engine load of 25%. This is probably caused by the high oxygen content in the combustion region for the LPM-diesel oil blends.
At the EGR rate of 12.8%, the BSFC for 5% LPM content in the fuel blends has lower or same values than that for the neat diesel oil at all engine loads. This is possibly caused by the presence of oxygen in the LPM substituting for the reduced oxygen in fresh air due to EGR application. The BSFC for the LPM content of 10% in the fuel blends is lower or same than that for the neat diesel oil without the engine load of 50%. However, the BSFC for the LPM content of 15% is increased at the same engine load compared with that for the neat diesel oil. This is caused by the retardation of reignition due to the high content of H2O in the LPM. H2O in the combustion region may inhibit reignition and extinguish the flame.
At the highest EGR rate, the values of BSFC for the engine operated with LPM in the fuel blends are higher compared with that of the neat diesel oil at the whole engine loads except for the engine load of 25%. This is probably caused by the combination effects of low heating value and high water content due to the presence of LPM. However, the BSFC for the engine operated with the D95LPM5 has smaller value compared with that of the neat diesel oil at the engine load of 25% due to the high oxygen content required for the complete combustion.
3.2 Effect of LPM on Brake Power
Figure 3 (a), (b) and (c) show the increase of brake power with the rise of engine loads for different proportion of LPM in the fuel blends at a given EGR rate. Generally, the presence of LPM in fuel blends reduces the brake power. This is because the heating value of LPM-diesel blends is lower compared with that of the neat diesel oil.
The present study was carried out for the variation of EGR rate in the range of 0 to 16.5%. For the case of no EGR (EGR rate of 0%), the addition of 5% LPM in proportion has no effect on the brake power compared with that of neat diesel oil at the engine load of 25% as can be seen in Figure 3 (a). However, the rise of LPM to 15% in the fuel blends reduces the brake power of 11.8% at the low load (25% engine load) and 7.6% at the full load (100% engine load). The smallest value of brake power is found for the fuel blends with LPM of 10% in proportion at the engine load of 75%.
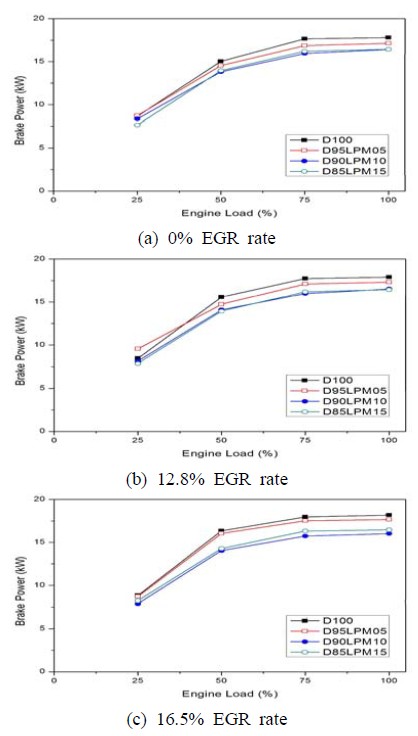
Effect of LPM proportion in the fuel blends on brake power for respective engine load at a given EGR rate
At the EGR rate of 12.8%, the brake power of 5% LPM in the fuel blends is bigger than that of neat diesel oil for the engine load of 25% as denoted in Figure 3 (b). This is caused by the reduction of oxygen from fresh air for combustion requirement due to the recirculated exhaust gas into combustion chamber. In the case of neat diesel oil, the reduction of oxygen results in the reduction of energy released by combustion. In the contrary, the presence of LPM with high content of oxygen is able to supply oxygen from fresh air to satisfy the combustion requirement. The brake power values are almost same for LPM contents of 10 and 15% in the fuel blends for all engine loads.
With the rise of EGR rate to 16.5%, the brake powers for the case of 5% LPM in the fuel blends seem the same value compared with that of neat diesel oil for the engine loads of 25 and 50% as shown in Figure 3 (c). However, the brake powers are reduced to average 3% in the case of 5% LPM in the fuel blends compared with that of neat diesel oil for the engine loads of 75 and 100%. In the cases of 10 and 15% LPM in the fuel blends, the brake powers of D90LPM10 are a little bigger than those of D85LPM15 for all the engine loads of experimental conditions.
3.3 Effect of LPM on Exhaust Gas Temperature
Figure 4 (a), (b) and (c) exhibit the effect of LPM on exhaust gas temperatures for the variations of engine load and LPM-diesel oil blend at the different EGR rates. The exhaust gas temperature is increased with the rise of engine load at all EGR rates. In the case of EGR application, the exhaust gas temperatures for the same engine load are discrepantly observed when the LPM content in the fuel blends is increased from 5 to 15%. In general, however, the addition of LPM in the fuel blends reduces the exhaust gas temperature due to the effects of methanol and H2O contents in the LPM. The first effect is resulted by the higher latent heat of vaporization of methanol (cooling effect).[17] The second effect is caused by the extinguishment of flame due to the presence of H2O.[21] It can be found that the changes of exhaust gas temperature are significantly affected by the addition of LPM in the fuel blends from 5 to 15%.
The exhaust gas temperature without the EGR rate is decreased as the proportion of LPM in the fuel blends is raised at the same engine load. In Figure 4 (a), the exhaust gas temperature is reduced to 14.1% when the LPM content is increased to 15% at the engine load of 25% and reduced to 19.9% at the full load compared to the neat diesel oil. By comparing with Figure 4 (a) and (b), however, the different phenomena can be seen by the proportion of LPM in the fuel blends. The exhaust gas temperature is almost same when LPM content is increased from 5 to 15% at the engine load of 25% at the EGR rate of 12.8%. Also, the exhaust gas temperatures are almost same for the engine operated with D95LPM5 and D85LPM15 at the engine loads of 25 to 75% as shown in Figure 4 (b). The exhaust gas temperature is reduced to 9.5% at the engine load of 25% and 16.8% at the full load for engine operated with the fuel blends of D90LPM10 compared to the neat diesel oil.
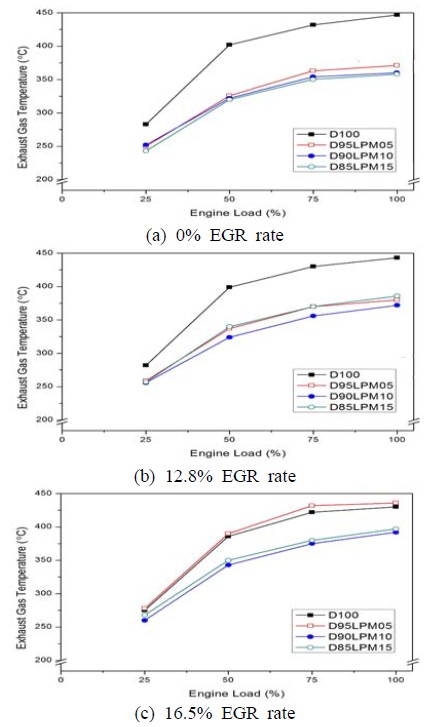
Effect of LPM on exhaust gas temperatures for the variations of engine load and LPM-diesel oil blends at a given EGR rate
When EGR rate is increased to 16.5%, the significant change of exhaust gas temperatures is observed as can be seen in Figure 4 (c). By comparing with the fuels of D95LPM5 and neat diesel oil, the differences of exhaust gas temperatures are considerably reduced. The exhaust gas temperatures operated with D95LPM5 for all the engine loads are found higher than those with the neat diesel oil. This can be explained that the reduction of oxygen due to the dilution effect in the combustion chamber by increasing EGR rate is replaced by the oxygen obtained from D95LPM5 fuel blends. This may result from the rise of combustion temperatures.
On the other hand, the exhaust gas temperatures of D90LPM10 for all the engine loads are lower than those of D85LPM15 in spite of applying EGR. This suggests that the oxygen effect contained in LPM is more significant than that reduced by increasing EGR rate. It can be seen, however, that the difference of exhaust gas temperature for the respective engine loads is getting small as the EGR rate is increased.
3.4 Effect of LPM on Brake Thermal Efficiency
The brake thermal efficiency indicates how the efficient energy in a fuel is converted into the mechanical energy output. The brake thermal efficiency for four kinds of fuel at a given EGR rate is increased when the engine load is raised from 25 to 75%, but decreased when the engine load exceeds 75% as shown in Figure 5 (a), (b) and (c). These are caused by the reduction of brake specific fuel consumption when the engine load is increased from 25 to 75% as can be seen in Figure 2. The brake thermal efficiency is also decreased at the full load resulting in the rise of brake specific fuel consumption. These phenomena are similar to those of the brake specific fuel consumption.
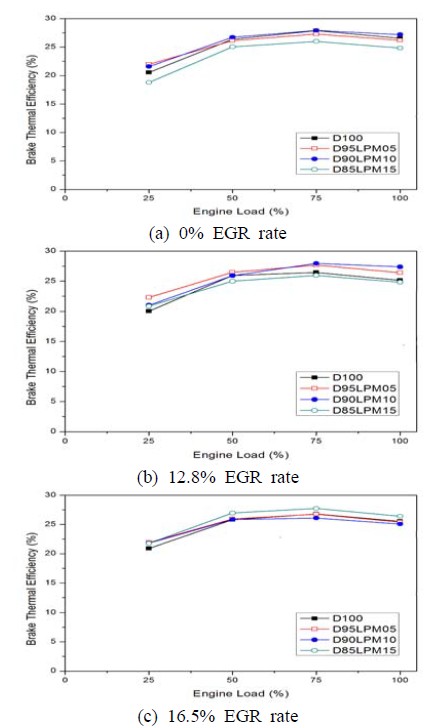
Effect of LPM on brake thermal efficiency for the variations of engine load and LPM-diesel oil blends at a given EGR rate
In the case of 0% EGR rate, the brake thermal efficiency for the neat diesel oil is 20.5% and 22% for D95LPM5 at the engine load of 25%, while it is reduced to 18.8% for D85LPM15 as shown in Figure 5 (a). The brake thermal efficiency for D90LPM10 is higher than that of the neat diesel oil at all engine loads. The brake thermal efficiency for D85LPM15 is lower than that of the neat diesel oil at all engine loads. This can be explained because the heating value for D85LPM15 is lower than that for the neat diesel oil and the amount of D85LPM15 is also considerably more than that of the neat diesel oil injected into the combustion chamber to obtain the same work output.
At the EGR rate of 12.8%, the brake thermal efficiency for the neat diesel oil is 20% and 24% for D95LPM5 at the engine load of 25% as denoted in Figure 5 (b). The brake thermal efficiency for D95LPM5 is higher than that of the neat diesel oil at all engine loads. This is probably caused by the presence of oxygen concentration in the LPM required for complete combustion although the oxygen due to dilution effect by applying EGR is reduced. The similar results are observed when the engine is operated with D90LPM10. However, the brake thermal efficiencies with D85LPM15 are reduced at the engine loads of 50 to 100% compared with the neat diesel oil.
At the EGR rate of 16.5%, the brake thermal efficiencies for D85LPM15 are higher than those for the neat diesel oil as denoted in Figure 5 (c). The differences of brake thermal efficiencies are not significantly observed at the rest of conditions for the engine operated with fuel blends.
3.5 Effect of LPM on Smoke Emission
The smoke opacity indicates the dry soot emission which is one among the main components of particulate matter.[18]Figure 6 (a), (b) and (c) denote the smoke opacity for the variation of engine load and fuel blend at the different EGR rates. Generally, the smoke opacity is increased as the engine load is raised. The addition of LPM in the fuel blends reduces the smoke opacity at the same engine load for all EGR rates. This can be explained by two reasons. First, the presence of methanol in the LPM fuel supplies the oxygen which reduces a formation probability of rich zone (locally high fuel-air ratio) and promotes the oxidation of soot nuclei in the fuel combustion.[22] Second, the presence of H2O in the LPM fuel reduces a cyclopentapyrene formation which indicates a large PAH formation and thus has the relative soot forming tendency. The increase of EGR rate results in the rise of smoke opacity for the same engine load and fuel blend as expressed in Figure 6. This is caused by the promotion of the smoke formation due to the reduction of oxygen concentration resulted from the dilution effect by applying EGR in the combustion region.
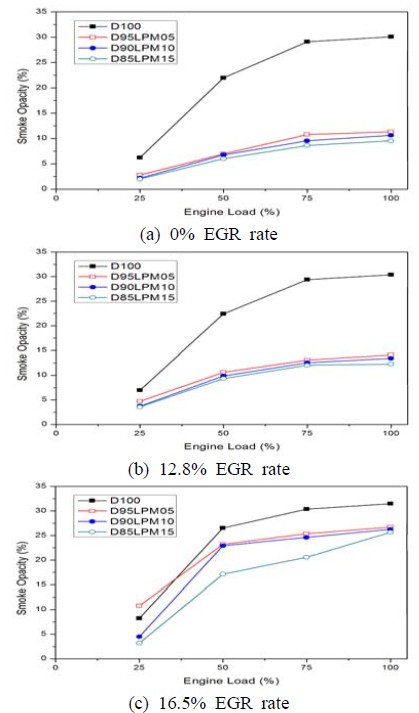
Effect of LPM on smoke opacity for the variations of engine load and LPM-diesel oil blends at a given EGR rate
In the case of 0% EGR rate, the smoke opacity for engine operated by the neat diesel oil is 6.2% and 1.98% for D85LPM15 at the engine load of 25%. It is also measured by 30.1% for the neat diesel oil and 9.5% for D85LPM15 at the full load. As mentioned earlier, the smoke opacity at all engine loads is decreased by the effect of LPM fuel as the proportion of LPM is increased from 5 to 15%. It can be seen from Figure 6 (b) with the EGR rate of 12.8% that the similar tendencies as Figure 6 (a) are presented. The smoke opacity for the neat diesel oil is 6.9% and 3.5% for D85LPM15 at the engine load of 25%, while it is measured by 30.4% for the neat diesel oil and 13.4% for D85LPM15 at the full load.
At the EGR rate of 16.5%, the smoke opacity for engine operated with D95LPM5 is higher than that for the neat diesel oil at the engine load of 25%. The smoke opacity has almost similar values at two types of fuel blends except for the engine operated with D85LPM15 at the half load. The differences of smoke opacity are not big at the full load when LPM content is increased from 5 to 15%. There is no significant influence of the smoke opacity at the full load when the LPM content is increased from 5 to 15% if the EGR rate is more than 15%.
4. Conclusions
The effect of low purity methanol on performance characteristics and smoke emission under four kinds of engine load was experimentally investigated using a direct injection diesel engine with EGR sy stem operated by the neat diesel oil and three kinds of fuel blends. The major results obtained from this work can be summarized as follows.
1) The brake specific fuel consumptions for almost all experimental conditions were raised with increasing LPM proportion in the fuel blends because the lower heating value of LPM is smaller than that of the neat diesel oil.
2) The presence of LPM in the fuel blends reduced the brake power for same engine load at all EGR rates compared with the neat diesel oil except for the blending fuel of 5% LPM proportion at the engine load of 25%, but the brake power for the LPM proportion of 5% in the fuel blends was similar to or higher than that for the neat diesel oil due to the supply of oxygen by the LPM.
3) The addition of LPM in the fuel blends reduced the exhaust gas temperature due to the effects of methanol and H2O contents in the LPM, but the exhaust gas temperatures with EGR application for the same engine load were discrepantly observed when the LPM content in the fuel blends is increased.
4) The influence of LPM proportion on the brake thermal efficiency was not constant owing to the combined effects of EGR rate and LPM.
5) The addition of LPM in the fuel blends reduced the smoke opacity at the same engine load for all EGR rates, and the increase of EGR rate brought to the rise of smoke opacity for the same engine load and fuel blends.
Acknowledgments
This paper is the result of a collaborative research between Department of Mechanical Engineering of Diponegoro University, Indonesia and Department of Mechanical Engineering for Production of Gyeongsang National University, Republic of Korea carried out by the support of the second phase BK 21 project (Advanced Mechanical and Aerospace Engineers Education Program).
Notes
References
- T. Kamimoto, and M. W. Bae, “High combustion temperature for the reduction of particulate in diesel engines”, SAE Paper 880423, p1-10, (1988).
-
T. Kamimoto, M. W. Bae, and H. Kobayashi, “A study on soot formation in premixed constant volume propane combustion”, Combustion and Flame, 75, p221-228, (1989).
[https://doi.org/10.1016/0010-2180(89)90039-4]
- J. Theis, and E. Gulari, “A LNT+SCR system for treating the NOx emissions from a diesel engine”, SAE Technical Paper 2006-01-0210, (2006).
- D. Chatterjee, T. Burkhardt, M. Weib, E. Tronconi, I. Nova, and C. Ciarde, “Numerical simulation of NO/NO2/NH3 reactions on SCRcatalytic converter model development and application”, SAE Technical Paper 2006-01-046, (2006).
- M. W. Bae, and Syaiful, “Numerical simulation on effect of heating temperature upon the gaseous flow in a SCR catalytic filter device”, SAE Technical Paper 2009-01-2628, (2009).
- M. W. Bae, W. C. Kang, and B. H. Song, “Reduction characteristics of nitrogen oxides emissions in LPG steam boiler with a Urea-SCR reactor”, Proceedings on KSAE 2011 Annual Conference and Exhibition (KSAE11-A0041), p224-228, (2011), (Electronic Form).
-
M. W. Bae, W. C. Kang, B. H. Song, and J. O. Bae, “Reduction characteristics of NOx emissions by optimal urea injection rate in a LPG steam boiler with Urea-SCR reactor”, 2012 International Conference on Systems and Informatics, p642-646, (2012).
[https://doi.org/10.1109/ICSAI.2012.6223079]
- K. Rajan, and K. R. Senthilkumar, “Effect of exhaust gas recirculation (EGR) on the performance and emission characteristics of diesel engine with sunflower oil methyl ester”, Jordan Journal of Mechanical and Industrial Engineering, 3, p306-311, (2009).
-
V. S. Yadav, S. L. Soni, and D. Sharma, “Performance and emission studies of direct injection CI engine in duel mode (Hydrogen diesel) with EGR”, International Journal of Hydrogen Energy, 37, p3807-3817, (2012).
[https://doi.org/10.1016/j.ijhydene.2011.04.163]
-
H. E. Saleh, “Effect of exhaust gas recirculation on diesel engine nitrogen oxide reduction operating with Jojoba methyl ester”, Renewable Energy, 34, p2178-2186, (2009).
[https://doi.org/10.1016/j.renene.2009.03.024]
-
M. Mani, G. Nagarajan, and S. Sampath, “An experimental investigation on a DI diesel engine using waste plastic oil with exhaust gas recirculation”, Fuel, 89, p1826-1832, (2010).
[https://doi.org/10.1016/j.fuel.2009.11.009]
-
D. Agarwal, S. K. Singh, and A. K. Agarwal, “Effect of exhaust gas recirculation(EGR) on performance, emissions, deposits and durability of a constant speed compression ignition engine”, Applied Energy, 88, p2900-2907, (2011).
[https://doi.org/10.1016/j.apenergy.2011.01.066]
- M. W. Bae, and J. K. Lim, “A study on effect of EGR upon fuel consumption rate and NOx emissions in diesel engines”, Transactions of the Korean Society of Automotive Engineers, 3(1), p76-88, (1995).
- M. W. Bae, “A study on the effects of recirculated exhaust gas on NOX and soot emissions in diesel engines with scrubber EGR system”, SAE Technical Paper 1999-01-3266, (1999).
- H. M Kim, M. W. Bae, and J. Y. Park, “The experimental investigations of recirculated exhaust gas on exhaust emissions in a diesel engine”, KSME International Journal, 15(11), p1588-1598, (2001).
- M. W. Bae, and Y. Mochimaru, “Characteristics of performance and exhaust emissions with a combined plasma EGR system in diesel engines”, Proceedings of the FISITA 2004 World Automotive Congress, p1-19, (CD) (2004).
-
M. Senthil Kumar, A. Ramesh, and B. Nagalingam, “An experimental comparison of methods to use methanol and Jatropha oil in a compression ignition engine”, Biomass and Bioenergy, 25, p309-318, (2003).
[https://doi.org/10.1016/S0961-9534(03)00018-7]
-
C. Sayin, “Engine performance and exhaust gas emissions of methanol and ethanol-diesel blends”, Fuel, 89, p3410-3415, (2010).
[https://doi.org/10.1016/j.fuel.2010.02.017]
-
Lei Zhu, C. S. Cheung, W. G. Zhang, and Zhen Huang, “Emissions characteristics of a diesel engine operating on biodiesel and diesel blended with ethanol and methanol”, Science of the Total Environment, 408, p914-921, (2010).
[https://doi.org/10.1016/j.scitotenv.2009.10.078]
- M. W. Bae, G. S. Ahn, J. H. Ha, and B. S. Lee, “Characteristics of injection and exhaust emissions in diesel engines with rice bran oil as an alternative fuel”, Proceedings of the International Conference on Fluid and Thermal Energy Conversion 2006, p103-1-103-13, (2006).
-
P. D. Teini, D. M. A. Karwat, and A. Atreya, “The effect of CO2/H2O on the formation of soot particles in the homogeneous environment of a rapid compression facility”, Combustion and Flame, 159, p1090-1099, (2012).
[https://doi.org/10.1016/j.combustflame.2011.10.002]
-
L. M. Pickett, and D. L. Siebers, “Soot in diesel oil jets: effects of ambient temperature, Ambient Density, and Injection Pressure”, Combustion and Flame, 138, p114-135, (2004).
[https://doi.org/10.1016/j.combustflame.2004.04.006]