
Composition of mixed gas with exhalation characteristics separated by dissolved gas separator with multi-stage passive mixer
Copyright ⓒ The Korean Society of Marine Engineering
This is an Open Access article distributed under the terms of the Creative Commons Attribution Non-Commercial License (http://creativecommons.org/licenses/by-nc/3.0), which permits unrestricted non-commercial use, distribution, and reproduction in any medium, provided the original work is properly cited.
Abstract
For underwater respiration, it is crucial to increase the amount of dissolved oxygen separated. Since the exhaled breath contains large amounts of oxygen, it is efficient to reuse the exhalation. However, to reuse the exhalation for respiration, the carbon dioxide contained in the exhalation must be reduced below a threshold value. In this study, by mixing a mixed gas with the characteristics of an exhalation with water, using a proposed passive mixer, large-sized bubbles are changed into several small-sized bubbles. By maximizing the contact area between the mixed gas and water, the dissolution of carbon dioxide was increased, and the carbon dioxide was effectively reduced when the mixed gas was separated through a separation device. When the 5 LPM of gas mixture and 40 LPM of water were mixed through the proposed passive mixing and separated through the separation device, the concentration of carbon dioxide was found to be less than 1%.
Keywords:
Multi-state mixer, Exhalation, Mixed gas, Composition, Separator, Carbon dioxide1. Introduction
To breathe underwater, we need underwater breathing apparatus. In the past, people would breathe underwater using an oxygen tank. Since the physical size of the oxygen tank is limited, people would need to come out of the water after a short period of underwater activity, replace the oxygen tank with a new oxygen tank, and re-submerge to continue the necessary work.
There are several advantages to being able to continuously collect the necessary oxygen in water. Shirtcliffe et al. showed the availability of dissolved oxygen [1]. A battery that consumed oxygen was placed in a box in the water; the material of the box was one that allowed oxygen to move. When the oxygen concentration in the box was measured, the oxygen concentration inside the box decreased over time for materials that did not move oxygen. When oxygen could move and water with sufficient dissolved oxygen was supplied, oxygen in the box initially decreased and then reached equilibrium. When oxygen was consumed by the battery, the oxygen concentration inside the box was lower than that of water, and the oxygen in the water moved into the box along the concentration gradient. This helped ensure that oxygen was continuously supplied and the oxygen concentration inside the box was balanced. A substance that was impervious to water and did not allow movement of oxygen was placed in the water in the form of a hollow box; when a situation that consumed oxygen inside occurred, the same amount of oxygen was supplied from the water as that consumed and the oxygen inside the box was balanced. This experiment suggests the possibility of underwater breathing. As the surface area of the box increases, more oxygen can expect to move.
Dissolved oxygen in water can be used, but a large amount of water is required because the amount of dissolved oxygen contained in water is small. The size of the device for handling this is also increased. To aid a person to carry and use it, a smaller size is beneficial.
To increase the amount of dissolved oxygen separated, Matsuda et al. suggested a carrier solution [2]. The use of a carrier solution had the effect of concentrating oxygen. Since the amount of dissolved oxygen was limited, the amount of dissolved oxygen separated per unit time was also limited. By first placing the carrier solution in contact with water to increase the oxygen concentration and then separating the dissolved oxygen from the carrier solution, the amount of dissolved oxygen separated per unit time could be increased.
Nagase et al. presented a carrier solution that responded to light [3]. The carrier solution had unique separation properties. The concentration could be controlled by adjusting the intensity of light without the need for a vacuum pump.
Velianti et al. suggested an increase in the separation rate of dissolved oxygen using magnetic materials [4]. Using magnetic properties helped increase the amount of dissolved oxygen separated. It was suggested that the amount of dissolved oxygen separated increased by coating the magnetic material on the surface of the separation element.
If the above method is used, the amount of dissolved oxygen separated increases, but a more effective method is to use dissolved oxygen for part of the required oxygen and exhalation for the remainder. A large amount of oxygen can be produced by using the components of exhalation well. I suggested an increase in the separation amount using a mixed gas simulating exhalation [5]. It could be observed that, when the amount of exhalation increased by mixing the mixed gas with water by a passive mixer, the separation amount increased. Moreover, when the amount of supplied water increased, the separation amount further increased under the same conditions. However, the amount of carbon dioxide contained in the exhalation is important if exhalation is to be used for underwater respiration. Reducing the amount of carbon dioxide contained in the gas mixture to use it for this purpose is crucial.
The proposed study shows that carbon dioxide contained in exhaled breath can be reduced. In other words, by effectively mixing the exhalation with water through a multi-stage manual mixer, the contact area between the exhalation and water can be maximized, and the amount of carbon dioxide contained in the exhalation can be reduced below an appropriate level. It was suggested that carbon dioxide was reduced by using a mixed gas having the characteristics of exhalation.
Since the size of the underwater breathing apparatus can be reduced, it is expected to be effectively used in the portable underwater breathing apparatus field. In addition, since it is possible to increase the residence time in the water, it is expected to be further utilized in fields requiring a long working time, such as underwater rescue, underwater exploration, and underwater work.
2. Experimental setup
The experimental apparatus consisted of the proposed manual mixer, inlet window, hollow fiber membrane, outlet window, vacuum pump, gas chromatography (GC), and mixed gas as shown in Figure 1. At this time, the proposed manual mixer consisted of six stages. The inlet window measured the condition through the manual mixer. The hollow fiber membrane was manufactured by LiquiCell. The outlet window was measured after the dissolved gas was separated by using a vacuum pump through the hollow fiber membrane. A vacuum pump was connected to the hollow fiber membrane and used to separate the dissolved gas. GC analyzed the component characteristics of the separated gas using a vacuum pump. Mixed gases having the characteristics of exhalation were prepared. The separation characteristics were investigated while increasing the flow rate by 1 LPM from 1 LPM to 5 LPM. At this time, the flow rate supplied increased by 10 LPM from 10 LPM to 40 LPM, and the separation characteristics were investigated. The mixed state of the feed water and the mixed gas before and after passing through the hollow fiber membrane was visualized. The composition characteristics of exhalation were investigated. The result of measuring the characteristics of exhalation by GC are shown in Figure 2(a). A mixed gas was prepared considering the characteristics of exhalation. The component characteristics of the prepared mixed gas are shown in Figure 2(b). There is inevitably a little difference in the composition of mixed gas for exhalation gas due to manufacturing conditions. The x-axis and y-axis represent time (min) and signal (μV), respectively. From the results of previous experiments, the first, second, and third component in Figure 2 represent oxygen, nitrogen, and carbon dioxide, respectively.
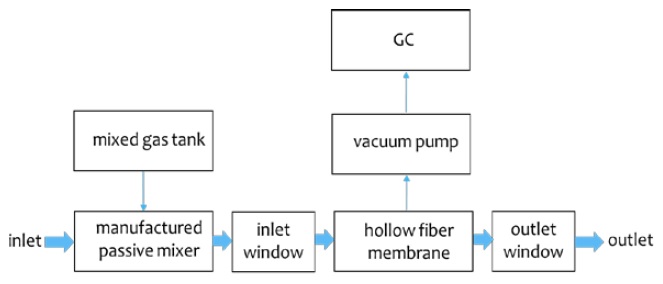
Outline (a) for the separation system of dissolved gases with small-sized bubbles and (b) for a passive mixer
3. Results and discussion
The composition characteristics of the gas separated by using a vacuum pump through the hollow fiber membrane were investigated using GC. The concentration of carbon dioxide in underwater breathing was closely related to that of carbon dioxide in the separated gas. The carbon dioxide content of exhaled breath is about 4.5%. The supplied gas mixture and the influent water are mixed in the proposed passive mixer. When mixing is effective, the concentration of carbon dioxide separated through the hollow fiber membrane reduces. When carbon dioxide is dissolved in water, the amount of carbon dioxide decreases. If the mixing is done evenly, the removal effect of carbon dioxide increases. This mixing state can be seen by visualizing the window before and after passing through the hollow fiber membrane. When the mixing is properly executed, a large amount of gases are separated through the hollow fiber membrane, and when the window at the outlet side is measured, the amount of bubble reduces significantly. However, if the mixing is not executed properly, air bubbles may remain without being removed by measuring the exit window after passing through the hollow fiber membrane. Figure 3 shows the mixed state of the mixed gas and the inlet before and after the hollow fiber membrane. Observation of the mixing state before passage through the hollow fiber membrane would show many small bubbles, whereas observation after passing through the hollow fiber membrane would show that the bubbles were almost separated. Therefore, mixing of influent water and mixed gas was effectively performed by using the proposed passive mixer.
Figure 4 shows that when the flow rate was 10 LPM, the amount of injected mixed gas increased to 1, 2, 3, 4, 5 LPM, the gas was separated using a hollow fiber membrane, and then the gas composition was measured using GC. It could be observed that the amount of carbon dioxide increased as the amount of mixed gas increased. Figure 5 shows the results of investigating the component properties of the separated gas while increasing the amount of mixed gas injected by 1 LPM from 1 LPM to 5 LPM when the flow rate is 20 LPM. Furthermore, as the amount of mixed gas increased, the amount of carbon dioxide increased. Figure 6 shows the component characteristics of the separated gas while increasing the amount of mixed gas injected when the flow rate is 30 LPM. The amount of carbon dioxide increased along with the amount of mixed gas. Figure 7 shows the carbon dioxide content of the separation gas according to the increase of the mixed gas when the flow rate was increased is 40 LPM. Moreover, when the amount of the mixed gas increased, the amount of carbon dioxide in the separated gas also increased. Importantly, when the flow rate increased, the amount of carbon dioxide in the separated gas decreased, even if the amount of the mixed gas was same. It is known that if the amount of carbon dioxide is less than 1%, it can be used for human respiration. In the case of 5 LPM of mixed gas mixed with 40 LPM influent, it could be seen by analyzing the characteristics of the separated gas that the amount of carbon dioxide was less than 1%. In other words, it indicates that the mixed gas of 5 LPM having the characteristics of exhalation can be reused for respiration. This has critical important implications in terms of using the underwater breathing apparatus. When the dissolved oxygen in water was purely separated and used, the amount of dissolved oxygen was limited; thus, a large amount of influent water was required with a corresponding device, which greatly increased the size and cost. Reuse of exhaled breath could play an important role in the miniaturization of aquatic respiration devices. However, it was necessary to reduce the carbon dioxide contained in the exhalation, and this study showed that the exhalation could be reused for respiration up to 5 LPM by using the proposed passive mixer. Moreover, the use of an underwater breathing apparatus that reuses the exhaled breath can greatly reduce the size of the apparatus compared with one that uses dissolved oxygen by separating it, making it portable and miniaturized.
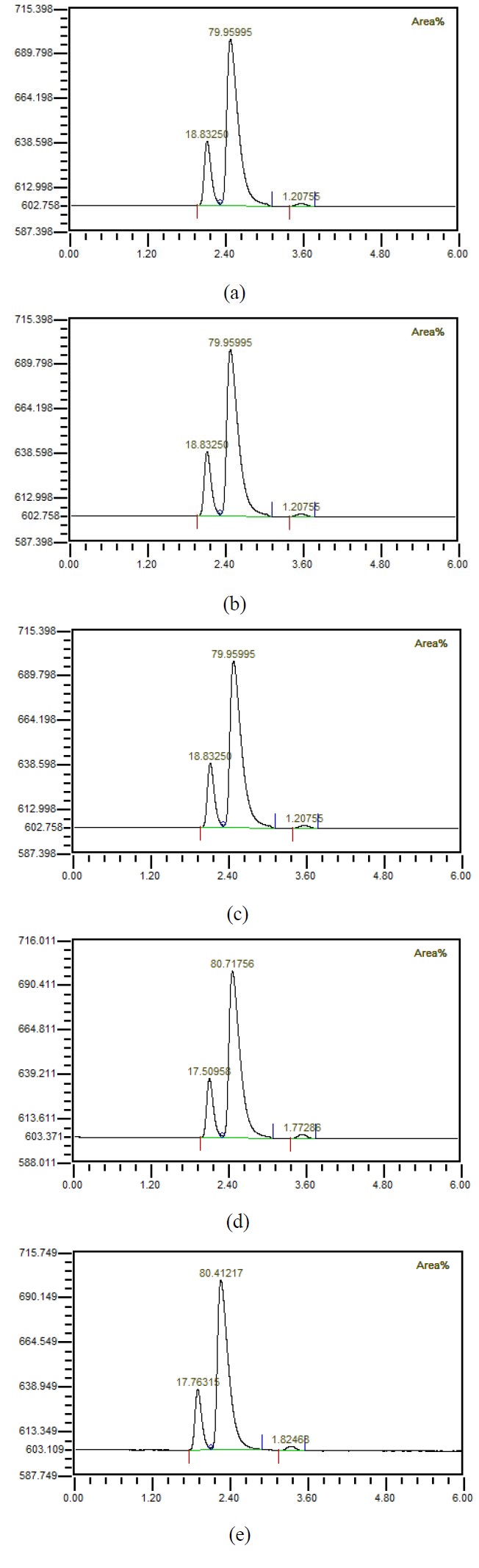
Composition of gases separated from water (a) with 1 LPM of prepared mixed gases, (b) with 2 LPM of prepared mixed gases, (c) with 3 LPM of prepared mixed gases, (d) with 4 LPM of prepared mixed gases (e) with 5 LPM of prepared mixed gases when 10 LPM water is mixed
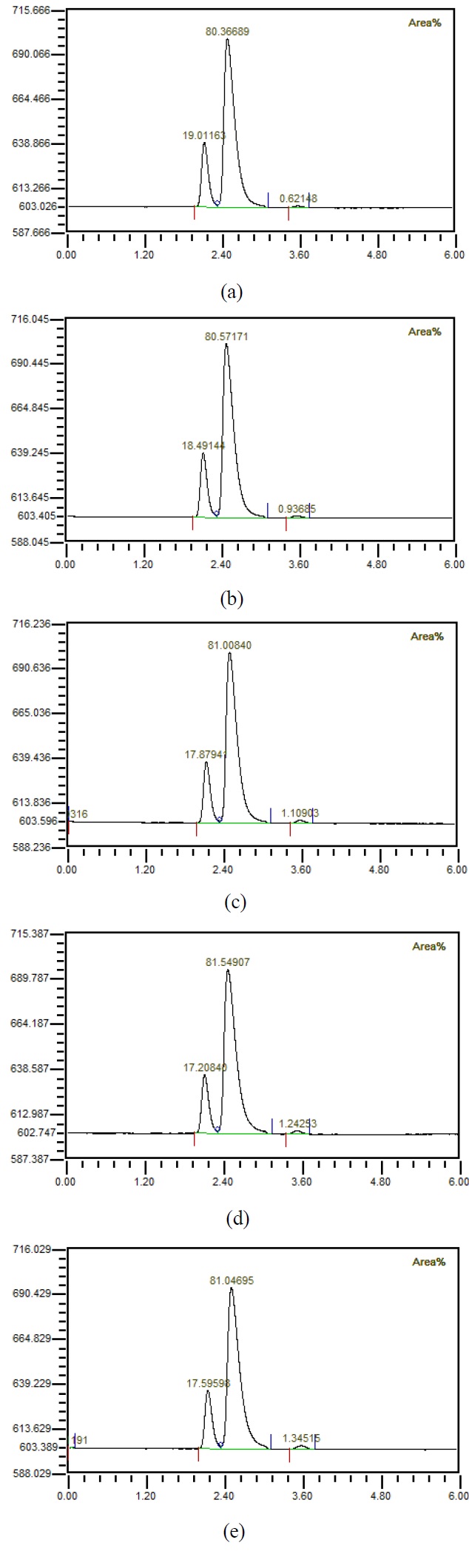
Composition of gases separated from water (a) with 1 LPM of prepared mixed gases, (b) with 2 LPM of prepared mixed gases, (c) with 3 LPM of prepared mixed gases, (d) with 4 LPM of prepared mixed gases, (e) with 5 LPM of prepared mixed gases when 20 LPM water is mixed
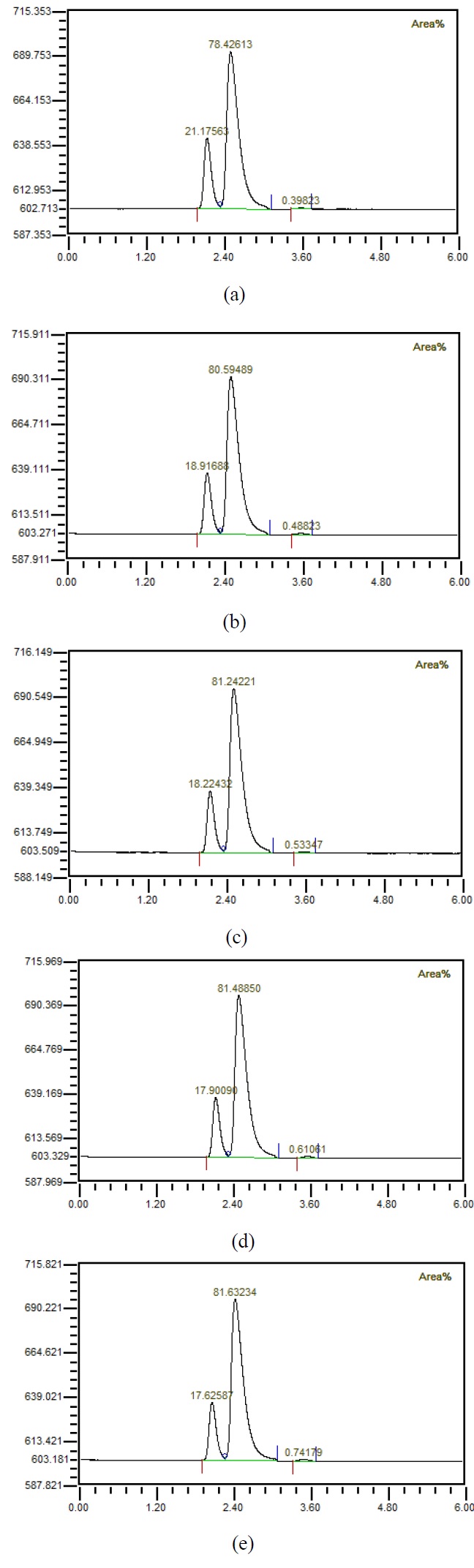
Composition of gases separated from water (a) with 1 LPM of prepared mixed gases, (b) with 2 LPM of prepared mixed gases, (c) with 3 LPM of prepared mixed gases, (d) with 4 LPM of prepared mixed gases, (e) with 5 LPM of prepared mixed gases when 30 LPM water is mixed
4. Conclusion
It was suggested that the concentration of carbon dioxide decreased compared to that contained in an exhalation when the mixed gas having the characteristics of exhalation was separated through the dissolved gas separation device after mixing using the proposed passive mixer. As the flow rate increased, the concentration of carbon dioxide decreased. When the flow rate was 40 LPM, it was shown that the concentration of carbon dioxide in the mixed gas separated through the separation device was 1% or less even if 5 LPM mixed gas was injected. If the concentration of carbon dioxide is less than 1%, it can be used for human respiration; thus, it is feasible to consider the possibility of underwater respiration using 5 LPM mixed gas corresponding to an exhalation. It may be possible to secure the large amount of dissolved oxygen required for underwater respiration by using exhalation. Therefore, it is easy to continue to secure oxygen required for underwater respiration using exhalation.
Acknowledgments
This research was supported by the Technology Development Project funded by the Korea Institute of Machinery and Materials (NK200H).
Author Contributions
Conceptualization, P. W. Heo; Methodology, P. W. Heo; Investigation, P. W. Heo; Resources, P. W. Heo; Data Curation, P. W. Heo; Writing-Original Draft Preparation, P. W. Heo; Writing-Review & Editing, P. W. Heo; Visualization, P. W. Heo; Supervision, P. W. Heo; Project Administration, P. W. Heo; Funding Acquisition, P. W. Heo.
References
-
N. J. Shirtcliffe, G. McHale, M. I. Newton, C. C. Perry, and F. B. Pyatt, “Plastron properties of a superhydrophobic surface,” Applied Physics Letters, pp. 104106-1-104106-2, 2006.
[https://doi.org/10.1063/1.2347266]
-
N. Matsuda, K. Sakai, T. Nakamura, and R. Majima, “Temperature-controlled enhancement of oxygen uptake from water using oxygen carrier solution,” Journal of Membrane Science, vol. 184, no. 1, pp. 17-26, 2001.
[https://doi.org/10.1016/S0376-7388(00)00602-5]
-
K. Nagase, F. Kohori, and K. Sakai, “Development of a compact artificial gill using concentrated hemoglobin solution as the oxygen carrier,” Journal of Membrane Science, vol. 215, no. 1-2, pp. 281-292, 2003.
[https://doi.org/10.1016/S0376-7388(03)00021-8]
-
Velianti, S. B. Park, and P. W. Heo, “The enhancement of oxygen separation from the air and water using poly(vinylidene fluoride) membrane modified with superparamagnetic particles,” Journal of Membrane Science, vol. 466, pp. 274-280, 2014.
[https://doi.org/10.1016/j.memsci.2014.04.043]
-
P. W. Heo, “Separation characteristics of gases from water including small sized unused gases through power-free mixing,” Journal of Advanced Marine Engineering and Technology, vol. 45, no. 6, pp. 442-446, 2021.
[https://doi.org/10.5916/jamet.2021.45.6.442]