
Separation characteristics of gases from water including small-sized unused gases through power-free mixing
Copyright © The Korean Society of Marine Engineering
This is an Open Access article distributed under the terms of the Creative Commons Attribution Non-Commercial License (http://creativecommons.org/licenses/by-nc/3.0), which permits unrestricted non-commercial use, distribution, and reproduction in any medium, provided the original work is properly cited.
Abstract
Dissolved oxygen can be used for underwater respiration. By injecting prepared mixed gases with the characteristics of exhalation into water and then separating the dissolved gasses through hollow fiber membranes, the separation amount of gases with oxygen can be increased. In this study, we propose a method of increasing the separation amounts of gases dissolved in water through a separator with hollow fibers by injecting prepared mixed gases with the characteristics of exhalation into water, and then separating the dissolved gases from the fluid passing through a passive mixer composed of multiple plates with evenly spaced small holes in the radial direction. After the prepared mixed gases were injected into the water, the mixed gases in the form of large bubbles were transformed into fine bubbles while passing through the fine-holed plates in multiple stages. The separation amount of gases through membranes was increased by enlarging the gas-liquid contact area by decreasing the bubble size. These results are expected to be beneficial for the development of portable underwater breathing devices owing to the high separation amount of dissolved gases in water by supplying mixed gases with fine bubbles through power-free mixing.
Keywords:
Unused gases, Small sized, Power-free mixing, Separation, Underwater1. Introduction
While humans can breathe freely in air, an additional device is required to assist with their breathing under water. Typically, humans breathe under water with the help of a compressed air reservoir. However, owing to the limited size of the reservoir, the time spent under water is limited.
Breathing under water using the oxygen dissolved in water can increase the duration of breathing under water. Some insects use the hair on their bodies to obtain oxygen from water [1]. Even if they are submerged under water, they can survive for a certain duration by extracting the oxygen dissolved in the water. The diameter, spacing, length, and contact angle of the hair vary from insect to insect. The interface between the water and gas is formed between the hairs, and the area of the interface changes depending on the depth of water. As the depth of the water increases, the area of the interface and the amount of gas exchange through the gas-liquid interface increase. However, at significantly large depths, the interface collapses. In other words, the characteristics of the diameter, spacing, and contact angle of these hairs limit the maximum depth of the interface. In contrast, when the depth is small, the underwater pressure decreases and the area of the gas-liquid interface decreases, thereby reducing the inflow of oxygen.
Fish can freely breathe in the dissolved oxygen in water through their gills, which have a structure that can effectively extract the dissolved oxygen in water. Gills are composed of arches and filaments, which contain numerous lamellas. When the gills come in contact with water through the lamellas, they extract oxygen and release carbon dioxide. The spacing between the lamellas is related to the power of 1/2 the lamellar length and the power of 1/6 the fish mass [2].
Theoretical models and experimental results of oxygen partial pressure, gas permeability, surface area, and membrane thickness have been presented in the literature [3]. As a result of the analysis of the models, when water without oxygen is supplied to the outside of a membrane in the presence of air inside the membrane, the oxygen concentration inside the membrane decreases. In this state, when oxygen-rich water is supplied to the outside of the membrane with oxygen-deficiency within the membrane, the oxygen concentration inside the membrane increases. When there is a difference in the oxygen concentration between the inside and outside of the membrane, oxygen movement can be observed owing to the concentration gradient. This suggests that as the thickness of the membrane decreases, the oxygen transport rate increases. Through experimentation, a trend similar to that of the theoretical model was observed. It was also observed that increasing the contact area of the interface caused the oxygen movement rate to increase.
By reducing the thickness of the membrane and increasing the contact area at the interface, the oxygen movement can be increased. However, because the amount of dissolved oxygen in water is limited, numerous membranes are required to supply the amount of oxygen needed by humans. To minimize the size of the device that can be used by humans, the use of exhalations is found to be effective [4].
In this study, we proposed a method to increase the separation amount of gases, including oxygen dissolved in water, by increasing the contact area between the gas bubbles and water through power-free mixing. Because water contains limited amounts of oxygen, large amounts of water and separation elements are required to increase the separation amount of dissolved oxygen. To supply the necessary amounts while carrying a person, there is a limit to the size of the separation system. Therefore, an efficient separation system is required, and the use of exhalation is considered as a suitable method. It is possible to minimize the size of the separation system by effectively using the oxygen contained in the exhaled breath. After supplying the prepared mixed gases with the characteristics of exhalation into the water, the fluid maximized the contact area between the gas and liquid by reducing the diameter of the mixed gases while passing through several disks with multiple holes in the radial direction. The separation amount of gases was increased by separating the gases through the separation device from water with an increased gas-liquid contact area.
The technology of using dissolved oxygen in water can be applied to fuel cells used as power sources in water [5]. This technology can also be applied to the measurement of radon, a radioactive substance that exists in a dissolved form in water [6].
2. Experimental
The experimental device is configured as shown in Figure 1(a), and it is composed of the prepared mixed gases, hollow fiber membranes, a mixer, a vacuum pump, and a measuring instrument. After injecting the prepared mixed gases into water, it was uniformly mixed while passing through the mixer and then supplied to the hollow fiber membranes. The gases contained in the water were separated through the hollow fiber membranes using a vacuum pump. The amounts of separated gases were measured by increasing the amount of mixed gases and supplied water.
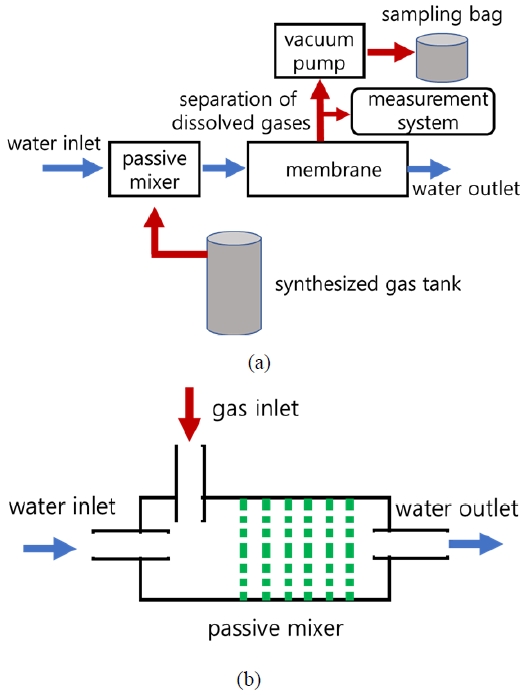
Outline for (a) the separation system of dissolved gases with small-sized bubbles and (b) a passive mixer
A passive mixer that did not use power was used, and as shown in Figure 1(b), the prepared mixed gases and water were mixed while passing through the plates with multiple holes in the radial direction in multiple stages. The hollow fiber membranes were acquired from a Liquicel company, and their specifications were listed in Table 1. The components of the prepared mixed gases were prepared by considering the characteristics of the exhalations. The prepared mixed gases were supplied to the passive mixer at 1, 2, 3, 4, and 5 L/min.
Tap water was supplied to the mixer by increasing the rate from 10 to 40 L/min. A self-made vacuum pump was used to separate the dissolved gases from water, and the flow rate and pressure of the separated gases were measured during operation.
3. Results and Discussions
Figures 2(a), (b), (c), (d), and (e) showed the amounts of gases separated when the prepared mixed gases at 1, 2, 3, 4, and 5 L/min were injected while water was supplied at flow rates of 10, 20, 30, and 40 L/min, respectively. Figures 3(a), (b), (c), (d), and (e) showed the vacuum pressure when the gases were mixed into the water at 1, 2, 3, 4, and 5 L/min for water flow rates of 10, 20, 30, and 40 L/min, respectively. Figures 4(a), (b), (c), and (d) showed the amount separated from the membrane and the vacuum pressure when the prepared mixed gases were mixed with water at 1, 2, 3, 4, and 5 L/min for water flow rates of 10, 20, 30, and 40 L/min, respectively.
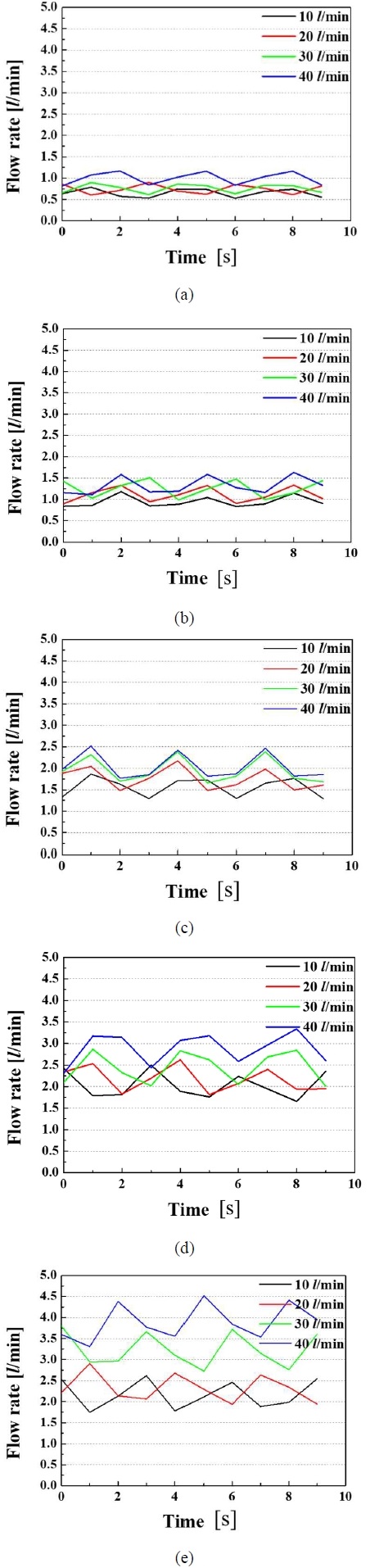
Flow rate of gases separated from water with (a) with 1 LPM, (b) 2 LPM, (c) 3 LPM, (d) 4 LPM, and (e) 5 LPM of prepared exhalation gases
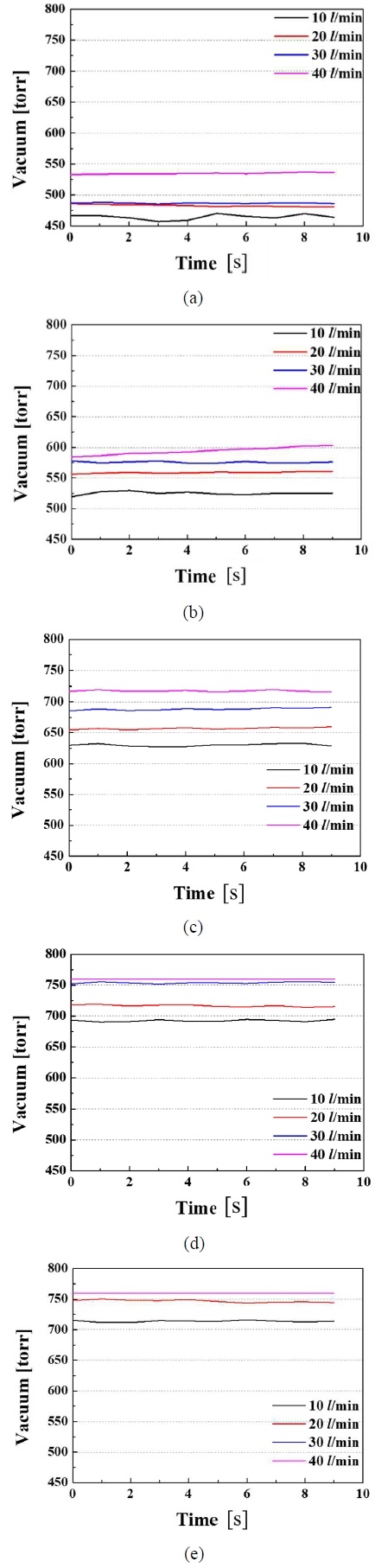
Vacuum pressure requested for gas separation from water mixed with (a) 1 LPM, (b) 2 LPM, (c) 3 LPM, (d) 4 LPM, and (e) 5 LPM of prepared exhalation gases
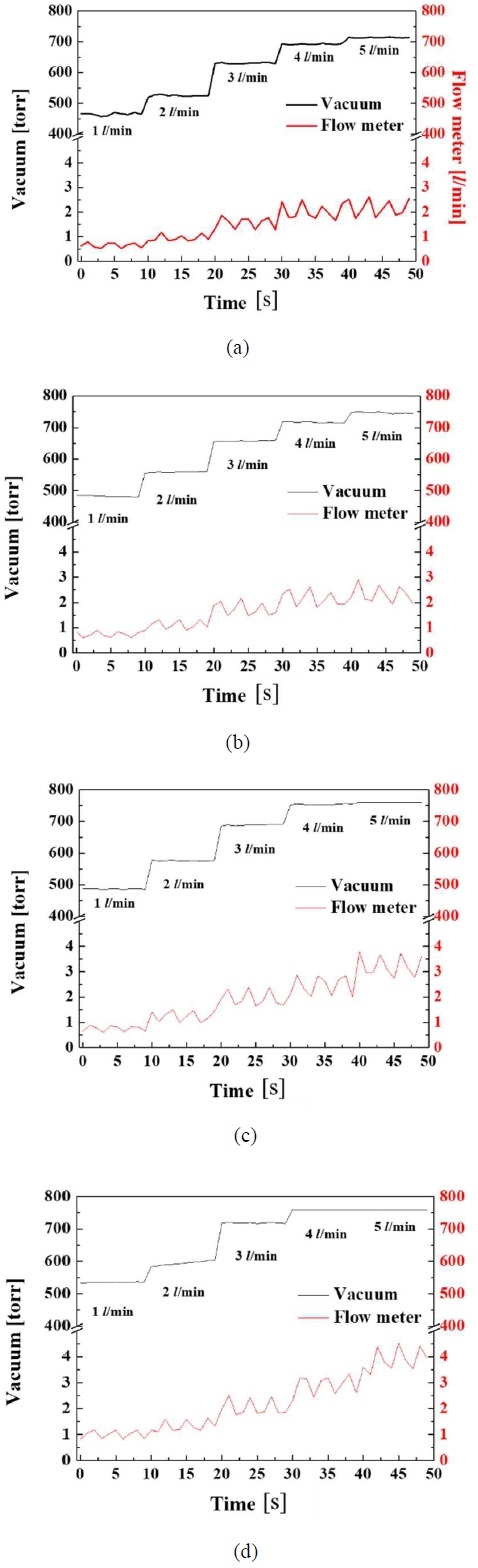
Vacuum pressure and flow rate of separation of gases for (a) 10 LPM, (b) 20 LPM, (c) 30 LPM, and (d) 40 LPM of supplying water
As shown in Figure 2, the amount of separated gases increased as the amount of prepared mixed gases injected into the water increased. When the amounts of water supplied were low, it could be observed that the separation of gases increased as the amount of the prepared mixed gases injected into the water increased, but the relative increase rate decreased. This meant that some gases were not separated from the separator and were discharged to the outside along with the discharged water. As the amount of incoming water increased, the amount of separated gases increased. The amount of separated gases was affected by the state of the prepared mixed gases and the injected water. When the separated amounts of gases were proportional to the amounts of gases in the prepared mixed gases, the bubbles were uniformly distributed under a certain size. When large-sized air bubbles were present, some air bubbles were not separated in part and were discharged together with water in the presence of air bubbles through the outlet. This meant that they were discharged to the outlet without separation. There was a loss in terms of the separation amounts. To increase the rate of separation, it is necessary to mix the gases with water in the form of small bubbles. Therefore, these results showed that the power-free mixer effectively produced small-sized bubbles and increased the contact area between the bubbles and water.
4. Conclusion
Prepared mixed gases were mixed with water and supplied to a membrane for separation. The prepared mixed gases dissolved in water were passed through several plates with multiple holes in the radial direction, appearing in the form of small bubbles. For the same amount of supplied water, as the amounts of prepared mixed gases increased, the amounts of gases separated through the membranes also increased. However, when the amount of supplied water was small, even when the prepared mixed gases were dissolved in water, the increase in the separation amount was limited. This implied that certain amounts of the prepared mixed gases were discharged to the outlet while remaining dissolved in water without being separated. Thus, an appropriate ratio between the amount of water and the amount of gas mixture to be dissolved needs to be considered. As the amounts of prepared mixed gases and supplied water increased, the amounts of separated gases also increased. Therefore, when water in form of small bubbles is supplied through passive mixing, the electric consumption can be reduced compared with that when mixing using a motor, and is thus favorable for the miniaturization of the underwater breathing apparatus.
Acknowledgments
This research was supported by the Technology Development Project funded by the Korea Institute of Machinery and Materials (NK200H).
Author Contributions
Conceptualization, P. W. Heo; Methodology, P. W. Heo; Investigation, P. W. Heo; Data Curation P. W. Heo; Writing-Original Draft Preparation, P. W. Heo; Writing-Review & Editing, P. W. Heo; Visualization, P. W. Heo; Supervision, P. W. Heo; Project Administration, P. W. Heo; Funding Acquisition, P. W. Heo.
References
-
M. R. Flynn and J. W. M. Bush, “Underwater breathing-the mechanics of plastron respiration,” Journal of Fluid Mechanics, vol. 608, pp. 275-296, 2008.
[https://doi.org/10.1017/S0022112008002048]
-
K. H. Park, W. J. Kim, and H. Y. Kim, “Optimal lamellar arrangement in fish gills,” Proceedings of the National Academy of Sceinces of the United States of America, vol. 111, no. 22, pp. 8067-8070, 2014.
[https://doi.org/10.1073/pnas.1403621111]
-
J. Lee, P. W. Heo, and T. Kim, “Theoretical model and experimental validation for underwater oxygen extraction for realizing artificial gills,” Sensors and Actuators A: Physical, vol. 284, pp. 103-111, 2018.
[https://doi.org/10.1016/j.sna.2018.09.071]
-
P. W. Heo, “Separation characteristics of separation devices using inlet water mixed with exhalation gases without a compressor,” Journal of the Korean Society of Marine Engineering, vol. 40, no. 9, pp. 842-846, 2016 (in Korean).
[https://doi.org/10.5916/jkosme.2016.40.9.842]
-
I. Ieropoulos, C. Melhuish, and J. Greenman, “Artificial gills for robots: MFC behaviour in water,” Bioinspiration Biomimetics, vol. 2, no. 3, pp. S83-S93, 2007.
[https://doi.org/10.1088/1748-3182/2/3/S02]
-
C. Lee, J. W. Bae, H. R. Kim, P. W. Heo, and H. S. Chae, “Radon in water measurement system based on the membrane-based air-water,” Radiation Measurements, vol. 121, pp. 54-60, 2019.
[https://doi.org/10.1016/j.radmeas.2018.11.006]