
Boiling CHF phenomena in water and FC-72
Extensive researches toward pool boiling characteristics have been widely investigated. However, the correct understanding of its boiling crisis by Critical Heat Flux (CHF) phenomenon during steady and transient heat transfer as a fundamental database for designing heat generation systems is still need to be clarified. The pool boiling CHFs were investigated to clarify the generalized phenomena of transition to film boiling at transient condition. The CHFs were measured on 1.0 mm diameter horizontal cylinder of platinum for exponential heat generation rates with various periods for saturated liquids at atmospheric pressure. The incipience of boiling processes was completely different depending on pre-pressurization. Also, the dependence of pre-pressure in transient CHFs changed due to the wettability of boiling liquids. The trend of typical CHFs were clearly divided into the first, second and third groups for long, short and intermediate periods, respectively. By the effect of pre-pressurization, the boiling incipience mechanism was replaced from that by active cavities entraining vapor to that by the HSN in originally flooded cavies.
Keywords:
Transient Boiling, Pool Boiling, Critical Heat Flux, Exponential Heat Input1. Introduction
The detailed understanding of generalized saturated and subcooled pool boiling CHF (Critical Heat Flux) phenomena in water and wetting liquids such as FC fluids (Fluorinert liquid), liquid nitrogen and liquid helium I, etc. is very important for energy conversion systems applied in land and marine environment. It is also important for the design and the safety assessment such as the high heat flux cooling systems using subcooled water pool boiling, the super-conducting magnets cooled by liquid helium and liquid nitrogen, the thermal control of microelectronic assemblies cooled by FC liquids for future super-computers.
The anomalous trend of CHF which increases, then decreases and again increases with decreasing of period, has been reported in previous papers [1]. Two main mechanisms of CHF exist depending on the exponential periods. One is due to the time lag of the hydrodynamic instability (HI) which starts at steady-state CHF on fully developed nucleate boiling, and the other one is due to the explosive-like heterogeneous spontaneous nucleation (HSN) which occurs at a certain HSN superheat in originally flooded cavities on a cylinder surface. Recently, the pool boiling CHF in several liquids was measured to investigate on the boiling behavior of transient phenomena [2]. It was confirmed that the vapor film behavior was significantly affected by the property and the wettability of the liquids. And the CHFs were divided into three regions for lower, intermediate and higher subcooling at a pressure resulting from HI, transition and HSN, respectively.
The role of spontaneous nucleation in originally flooded cavities on the cylinder surface called HSN is dealt with in this paper. The HSN phenomenon occurs when a new phase appears at an interface or a boundary rather than in the bulk fluid similar to the homogeneous spontaneous nucleation [3]. It has been clarified by Sakurai et al. [4] that CHFs due to steady and transient heat generation rates on the cylinder surface at certain conditions in water are determined by the explosive-like HSN: it occurs at the HSN surface superheat in originally flooded cavities on the cylinder surface. The HSN phenomenon was observed on the cylinder surface in previously degassed water before each experimental run by high pressure of 5 MPa for a while: the incipience of boiling from active cavities previously entraining vapor was replaced to that resulting from the HSN in originally flooded cavies.
The objective of this work is to clarify an effect of the pre-pressurization at a pool boiling CHF, and to make clear the generalized phenomena at transient CHF depending on the wettability of boiling liquids. As a continuation of previous work [2], boiling heat transfer processes on a horizontal cylinder in water (dealt with non-wetting liquid here) or highly wetting liquid such as FC-72 due to exponentially increasing heat input with various periods were measured for saturated liquids at atmospheric pressure. The present study is to investigate the transition phenomena to film boiling by the photographic approach on the vapor bubble and vapor film behavior on cylinder surface by using a high-speed video camera. The final purpose of this work is to accumulate the CHF database to clarify the generalized CHF phenomena in various liquids.
2. Experiment Apparatus and Method
The experimental apparatus is shown in Figure 1. The experimental apparatus consists of a boiling vessel, a horizontal cylinder of experimental heater, a pressurizer, a control device of heat generation rate, a data measurement and processing system, and a high-speed video camera. The boiling vessel with inspection windows is made of stainless steel having an inner diameter of 200 mm and a height of 600mm. The test heater is made of platinum wire having a diameter of 1.0 mm, which is horizontally mounted in the vessel.
The experimental heater is heated electrically by a direct current source controlled by a computer as it is increased in an exponential function with time. The analogue computer computes the instantaneous mean temperature of the cylinder and it cuts off the power supply when the calculated mean temperature reaches a preset value by using the burnout detector. The output voltages of the double bridge circuit, together with the voltage drops across the potential taps of the cylinder and across a standard resistance, were amplified and passed through analog-to- digital (A/D) converters installed in computer. These voltages were simultaneously sampled at a constant time interval that was changed depending on period. The fastest sampling speed of the A/D converter is 5 ms/channel. The average temperature between the potential taps was measured by resistance thermometry using the cylinder itself. The heat generation rate was determined from the current to the cylinder and the voltage difference between potential taps on the cylinder. The surface temperature was obtained by solving the conduction equation in the cylinder under the conditions of the average temperature and heat generation rate. The CHF was determined at a start point where the average temperature rapidly increases up to the preset temperature that is lower than the actual burnout temperature of a platinum wire. The uncertainties are estimated to be within ±1 percent in the heat generation rate, ±2 percent in the heat flux and to be within ±1 K in the cylinder surface temperature.
The heat generation rate Q was raised with exponential function as follows;
where, Q0is initial heat generation rate, W/m3 and t is time, s. τ is period, s: the e-fold time corresponding to heat generation rate with the exponential increasing rates from quasi-steady to rapid ones. The heat flux q was calculated by the following equation for heat balance.
where, D, ρh, ch and Ta are the diameter, density, specific heat and the average temperature of cylinder, respectively. The surface temperature can be calculated from unsteady heat conduction equation of the next expression by assuming the surface temperature around the test heater to be uniform.
Boundary conditions are as follows,
where, Q (W/m3)is the internal heat generation rate, T (K) is the temperature inside the cylinder, a = k /ρc (m2/s) is thermal diffusivity, and k (W/mK) is thermal conductivity.
The experiment was carried out as follows. First, the experimental liquids were degassed by keeping it boiling for 30 minutes at least in the auxiliary tank. Vapor was recovered to the pool with a water- cooled condenser. The liquid was fully filled in the boiling vessel with the free surface only in the pressurizer and sub tank. Liquid temperatures in the boiling vessel and in the pressurizer were separately controlled to realize the desired saturated and subcooled conditions. Each of heat flux and surface superheat was calculated by the data processing system with time. The heat input was raised with exponential function, Q= Q0exp(t/τ). Q0 is initial heat generation rate, W/m3 and t is time, s. τ is period, s: the e-fold time corresponding to heat generation rate with the exponential increasing rates from quasi-steady to rapid ones. Table 1 shows the experimental condition.
3. Experiment Results and Discussion
In this study, heat transfer processes for periods longer than 10 s are considered as quasi-steady-state processes, because the non-boiling region in such processes agrees well with the natural convection curve, and all CHFs measured for the heat inputs with periods longer than 10 s are almost the same.
3.1 Effect of Pre-pressurization on Heat Transfer Processes in Water
Pre-pressurization was also taken consider to a large potential of nucleation sites dissipation on the surface cavities then assumed trigger a spontaneous nucleation of boiling process. According to a series of experiment under atmospheric pressure, the surface superheat at incipience of boiling, ΔTin, for lower pre-pressures showed the effect of pre-pressurization due to active cavities on cylinder surface. But the constant ΔTin values were measured for higher pre-pressures [6][7] as shown in the Figure 2.
The heat transfer processes with various pre-pressurization with the exponential heat inputs for the period of 20 s (means quasi-steadily increasing one) at atmospheric pressure for saturated condition in water are shown on the graph of heat flux q versus surface superheat ΔTsat in Figure 3. It can be found that the incipience of boiling processes is completely different from each other, but the CHF values are almost same regardless of the value of pre-pressures. The surface superheat at incipience of boiling for pre-pressure of none, 1.2 MPa and 5 MPa results the ΔTin of 16 K, 25 K and 40 K, respectively.
The heat transfer processes with two kinds of pre-pressurization with the exponential heat inputs for the period of 100 ms meaning highly increasing one at atmospheric pressure for saturated condition in water are shown on the graph of heatflux q versus surface superheat ΔTsat in Figure 4. It can be found that the incipience of boiling processes and boiling curves are completely different from each other depending on pressurization. In the case of pre-pressurized one, it shows the transition from non-boiling to film boiling directly caused by HSN surface superheat. By the effect of pre-pressurization, the boiling incipience mechanism was completely changed because it became previously degassed water before the experiment run. Photographs of vapor film behavior will be shown later. The surface superheat at incipience of boiling for prepressure of none and 5 MPa results the ΔTin of 20 K and 80 K, respectively.
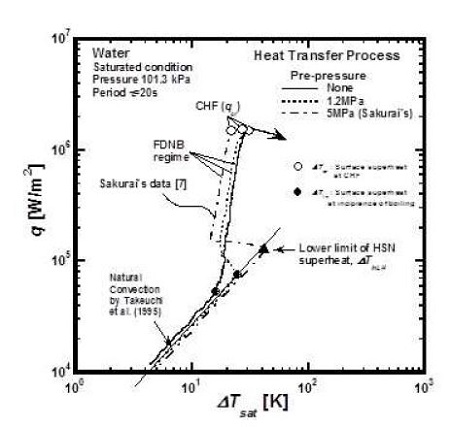
Typical heat transfer processes from non-boiling to fully developed nucleate boiling (FDNB) depending on pre-pressurization
3.2 Effect of Pre-pressurization on Heat Transfer Processes in FC-72
The surface superheats at incipience of boiling, ΔTin, versus pre-pressure were measured under saturated FC-72 at atmospheric pressure for the period of 20 s (means quasi-steadily increasing heat input) as shown in the Figure 5. A boundary line at Tin≅ 56 K divides two areas. The above area of the line represents the direct transition (ΔTin=ΔTcr) due to HSN as mentioned before. It gives an elevated typical of ΔTin by increase of pre-pressure. The below area shows the surface superheat at boiling incipience due to active cavities on the cylinder surface. It could find the potentially active cavities required for boiling initiation considerably exists on the cylinder surface at a range of pre-pressures from 0 MPa to 1 MPa,. As a result, at the pre-pressure of 0 MPa (without pre-pressruization), ones appear no exist of ΔTin that of due to HSN, on the contrary, ΔTin were observed fully occur due to HSN at 1.2 MPa.
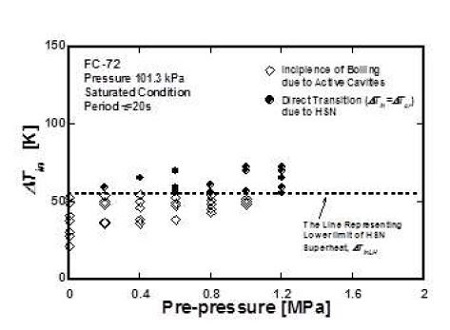
Incipient boiling superheat vs. pre-pressure under saturated FC-72 at atmospheric pressure for period of 20 s
Figure 6 shows the heat transfer processes with and without pre-pressurization in FC-72. The boiling curve without pre-pressurization is shown with a solid line: heat flux, q, increases along the natural convection curve at first and after the incipience of boiling at a surface superheat of 30 K, the surface superheat rapidly decreases, and the transition to fully developed nucleate boiling occurs and reaches the CHF point. On the other hand, in the case of pre-pressurized one as shown with a dashed line, the CHF point is due to the direct transition from single-phase conduction regime to film boiling; the value qin is equal to qcr. The boiling occurs at a surface superheat point of 60 K, which is higher than that of none pre-pressured one. It is considered that the direct boiling transitions occur due to the levitation of liquid on the cylinder surface by the explosive-like HSN in originally flooded cavities without contribution of the active cavities entraining vapors [6]-[8].
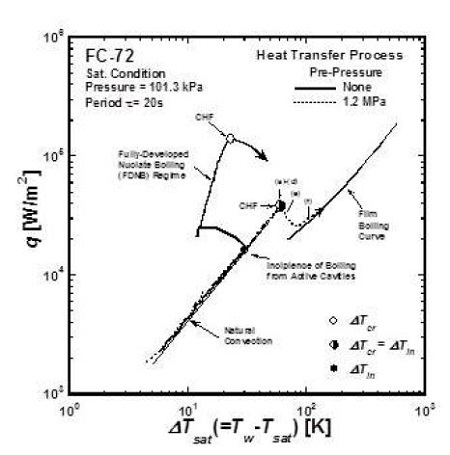
Transitions from non-boiling to film boiling or to fully-developed nucleate boiling (FDNB) caused by exponential heat inputs in saturated FC-72 at pressure of 101.3 kPa. Photographs shown in Figure 9 was taken at points of (a) to (f) on the graph.
Here, it turns out that both superheats at incipience of boiling completely changes because of pre-pressurization. That is, it becomes there is no active cavities entraining vapor by applying pre-pressure, and it is hard for incipience of boiling. Moreover, the difference of the CHFs on each condition has come out, and it is important for the database in the design of various kinds of heat flux apparatus.
By the way, the transition processes can be classified into three principal groups. The first group is, the CHF occurred through a fully developed nucleate boiling as shown in the Figure 3 and Figure 6 in the case of none pre-pressured one. And the direct transition to film boiling without nucleate boiling belongs to the second group as shown in the Figure 4 in the case of the 5 MPa pre-pressured one, and in the Figure 6 in the case of the 1.2 MPa pre-pressured one. Finally there exists the third group for intermediate one between first and second groups.
3.3 Quasi-steady-state CHF under saturated condition in FC-72
The steady-state CHFs, qcr,sat, measured under saturated condition at a wide range of pressures, P, from atmospheric pressure up to 1278 kPa are shown in Figure 7. The corresponding curve obtained from the saturated CHF correlation based on hydrodynamic instability (HI) model derived by Kutateladze [9] in Equation (1) was plotted for comparison, with the proportional coefficient K1 of 0.17.
The CHFs measured almost agree with the corresponding curve as shown in the figure, except ones at high pressures above 1.0 MPa which give a slight higher of rate than those derived from Equation (1). Namely, it is assumed that the transition occurs due to the physical model of a new CHF mechanism in the area near the critical pressure (1.8 MPa) of FC-72. It is from the explosive-like heterogeneous spontaneous nucleation (HSN). Further consideration in detail needs.
3.4 Photographs of Vapor Film Behavior in Water and FC-72
In this section, the explosive boiling due to HSN is dealt with, instead of the typical nucleate boiling caused by active cavities. Figure 8 shows the photographs for with a period of 100 ms at a pressure of 101.3 kPa at the corresponding points shown in Figure 4. Figure 8(a) is the photograph at point (a), which is the onset of boiling. Figure 8(b) is the photograph after a time passage of 5 ms from point (a). After the onset of boiling as photo no. (a) and (b), it shows a large spherical vapor bubble due to the HSN which is formed rapidly within 5 ms. After that, it covers the whole cylinder surface with large vapor tube. This is typical behavior of direct transition due to the H S N.
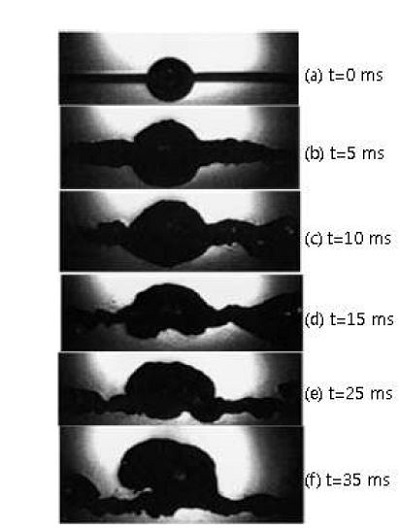
Vapor film behavior during direct transition to film boiling at atmospheric pressure in saturated water with pre-pressurization (Sakurai et al. (2000)).
On the other hand, Figure 9 shows the photographs for the pre-pressurization at the corresponding points shown in Figure 6. Figure 9(a) is the onset of boiling on the cylinder. The (b) taken at 1 ms after the first one shows a vapor tube due to the explosive-like HSN in flooded cavities, and it covers the whole cylinder surface by the large vapor tube as shown (c)-(d). The (e) show the vapor bubbles collapse from the boiling initiation bubbles. Then, large vapor bubbles are broken away from the large vapor film by buoyancy force and move upward. The temperature difference of the surface superheat between corresponding to Figures 9(a) to 9(d) is almost the same. After detachment of the large vapor bubbles, solid-liquid contacts occurs, and then new thin vapor film with the Taylor unstable wave on the upper part of the vapor-liquid interface covering the cylinder is formed by the explosive-like HSN on the places of solid-liquid contact and thin film boiling. At this moment the surface temperature starts increasing rapidly as a result of heat transfer deterioration. As shown in Figure 9(f), the behavior of vapor-liquid interface in film boiling on the cylinder similar to that for steady-state film boiling on the cylinder is clearly observed after the detachment of large vapor bubbles.
4. Conclusions
The pool boiling CHFs were investigated to clarify the generalized phenomena of transition to film boiling at transient condition.
(1) The incipience of boiling processes was completely different depending on the pre-pressurization and the wettability of boiling liquids.
(2) By the effect of pre-pressurization, the boiling incipience mechanism was replaced from that by active cavities entraining vapor to that by the HSN in originally flooded cavies.
(3) The direct transition without nucleate boiling to film boiling was explained by the HSN.
Acknowledgments
This paper is extended and updated from the short version that appeared in the Proceedings of the International symposium on Marine Engineering and Technology (ISMT 2013), held at BEXCO, Busan, Korea on October 23-25, 2013.
References
-
A. Sakurai, and K. Fukuda, “Mechanisms of subcooled pool boiling CHFs depending on subcooling, pressure, and test heater configurations and surface conditions in liquids”, Proceedings of The American Society of Mechanical Engineers International Mechanical Engineering Congress & Exposition, p1-13, (2002).
[https://doi.org/10.1115/IMECE2002-39066]
- J. Park, K. Fukuda, and Q. Liu, “Steady-state and transient CHFs in various liquids due to exponentially increasing heat inputs”, Proceedings of The 3rd Asian (OCMT-QDU) Cooperative Technology and Education Conference, p5-10, (2008).
- B. P. Avksentyuk, and N. N. Mamontova, “Characteristics of heat transfer crisis during boiling of alkali metals and organic fluids under free convection conditions at reduced pressure”, Prog. Heat Mass Transfer, 7, p355-362, (1973).
- A. Sakurai, M. Shiotsu, and K. Hata, “New transition phenomena to film boiling due to increasing heat inputs on a solid surface in pressurized liquids”, Instability in Two Phase Flow Systems, HTD-260(H00838), p27-39, (1993).
-
J. Y. Chang, S. M. You, and A. Haji-Sheikh, “Film boiling incipience at the departure from natural convection on flat, smooth surfaces”, Journal of The American Society of Mechanical Engineers, 120, p402-409, (1998).
[https://doi.org/10.1115/1.2824264]
- A. Sakurai, M. Shiotsu, and K. Fukuda, “Pool boiling critical heat flux on a horizontal cylinder in subcooled water for wide ranges of subcooling and pressure and its mechanism”, Proceedings of the American Society of Mechanical Engineers Thirty-first National Heat Transfer Conference, HTD-326, p93-104, (1996).
-
A. Sakurai, “Mechanisms of transitions to film boiling at CHFs in subcooled and pressurized liquids due to steady and increasing heat inputs”, Nuclear Engineering and Design, 197, p301-356, (2000).
[https://doi.org/10.1016/S0029-5493(99)00314-3]
- K. Fukuda, M. Shiotsu, and A. Sakurai, “Transient pool boiling heat transfer due to increasing heat inputs in subcooled water at high pressures”, Proceedings of the 7th International Meeting on Nuclear Reactor Thermal Hydraulics, Saratoga Springs, 1(NUREG/CP-0142), p554-573, (1995).
- S. S. Kutateladze, “Heat transfer in condensation and boiling”, AEC-tr-3770, USAEC, (1959).