
Hazard identification (HAZID) of LNG dual-fueled ships operating between the Korean port of Busan and the Iranian port of Bandar Abbas
Copyright ⓒ The Korean Society of Marine Engineering
This is an Open Access article distributed under the terms of the Creative Commons Attribution Non-Commercial License (http://creativecommons.org/licenses/by-nc/3.0), which permits unrestricted non-commercial use, distribution, and reproduction in any medium, provided the original work is properly cited.
Abstract
This study investigates the scope of hazard identification (HAZID), focusing in particular on analysis of the causes and consequences of hazardous scenarios for overall LNG fuel systems. The main categories of hazard scenarios are: fire and explosion initiating from LNG systems, fire and explosion not LNG-initiated, dropped objects, collisions, grounding, foundering, and occupational accidents. The main goals of this study are: identifying potential hazards to be addressed in further concept risk analysis assessment; providing input on possible solutions to be assessed in the vessel concept development work; and providing input to external communication from the vessel/project, clearly describing how potential risk should be identified and addressed through the vessel/project. The purpose of the HAZID in our study is to identify safety hazards that may represent risks to crew and third parties, e.g., maintenance personnel, yard workers, and other ships during operation.
We identified 34 hazards and made 18 recommendations with a comprehensive summary in terms of design and operation, which covered a wide-range of design and operation topics with the division in high prioritization. The main result from the HAZID shows that the estimated HAZID increase is mainly due to the presence of the LNG tank and its effect on the risk from fire/explosions due to ship collision. The HAZID results confirm that there are no major HSE showstoppers to perform construction and conversion on vessels using dual-fuel. The main selection criterion is the potential design, worst-case scenario for location of LNG tank below accommodation, and technical and operational capabilities in conducting such a HAZID study and investigations. Several significant gaps in mandatory regulations, standards, guidelines, or of relevant organizations beyond mandatory regulations were identified and addressed.
Keywords:
Liquefied natural gas, Hazard Identification (HAZID), The IMO interim guideline MSC.285(86), IGF code, LNG-dual - fueled ship, Fire and explosion, Hazard and effects1. Introduction
Utilization of liquefied natural gas (LNG) is rapidly increasing and it is seen as a viable alternative to heavy fuel oils/marine diesel oil owing to several factors, such as its properties, economic and environment circumstances, and its business reaching a mature phase [1]-[6].
To cope with the demand of the LNG Market with flexibility, this study has novelly assessed and developed the Hazard Identification (HAZID) system that addresses all areas that need special consideration. For the usage of the natural gas fuel-low flashpoint fuel to become a global fuel choice, it is essential that gaps and barriers on national and international regulations and standards are assessed and evaluated to promote safety and minimize the risk to the ship, its crew, and the environment, and conform to the regulations in the new energy sector, ensuring that any risks, gaps and barriers arising from the use of Natural Gas-fueled engines affecting the integrity of the vessel's main safety functions are addressed [11]-[18][43].
The first version of the international Code of safety for ships using Gases or other low-flashpoints (IGF Code) was adopted by resolution MSC.391(95), which was implemented on 1 January 2017. This first version of the IGF Code addresses only LNG (methane). Other low flashpoint fuels are being considered and amendments are made to the IGF Code as necessary.
The IMO has been tasked to develop the second version of the code, addressing methyl/ethyl alcohol and other low-flashpoint fuels such as low-flashpoint diesel.
The IMO Interim Guidelines on Safety for Natural Gas-Fuelled Engine Installations in Ships MSC 285(86) (Interim Guideline) and IGF code (International Code of Safety for Ships using Gases or other Low Flashpoint Fuels) requires that a ship using an alternative fuel demonstrates by risk analysis that the safety level is equivalent to that of a conventional oil-fueled ship [8]-[10]. Therefore, the aim of this study is to perform a HAZID to meet the requirements of the IMO Interim Guideline and IGF Code. The goal of these Interim Guidelines is to provide criteria for the arrangement and installation of machinery for propulsion and auxiliary purposes, using natural gas as fuel, which will have an equivalent level of integrity in terms of safety, reliability, and dependability as that which can be achieved with new and comparable conventional oil-fueled main and auxiliary machinery. It is assumed that the vessel is powered by on gas while performing on-loading and off-loading operations of containers.
This analysis represents the HAZID concept and is based on innovative thinking, maritime industrial experience, and the design concept of the vessel; the vessel is a typical container ship operating between the Korean port of Busan and the Iranian Port of Bandar Abbas [19]-[41][46].
2. Vessel Design Concept
The vessel design data, which complies with IMO Interim Guidelines on Safety for Natural Gas Fuelled Engine Installations in Ships MSC 285(86), is shown in Table 1. The deadweight is approximately 81,000 ton on a draft of 13.20 m. The design speed (suitable service speed) is approximately 21 knots. The endurance for using gas fuel is set to 11, 200 NM (Nautical mile) for one round trip between Iran/Bandar Abbas and Korea/Busan. In addition, the endurance for emergencies (using diesel oil) is estimated to be 5,600 NM.

Design data of LNG-fueled 8,000 TEU container ship - ship design data compared to the 8,000 TEU reference ship
The accommodation is arranged in the forepart of the vessel to facilitate and increased number of containers on deck whilst remaining in accordance with IMO visibility requirements. The safety barriers around the bunkering station (i.e., ventilation and shielding, drip trays, protection against overfilling, emergency shutdown (ESD) system and other safeguards) are aimed at reducing the likelihood of an accidental spill. Further, they minimize the consequence in the event of spilled LNG, where safety barriers for the “Room for LNG Tanks” (i.e., ventilation, gas detection and fire]/ extinguishing, independent bilge system, LNG tank support, and anti-rolling and anti-pitching chocks) are designed and comply with IMO Interim Guidelines on Safety for Natural Gas-Fuelled Engine Installations in Ships MSC 285(86) [7]-[9].
The vessel has one room for the LNG tank around the mid ship, under the accommodation area, as shown in Figure 1. The general arrangement is shown in Figure 2.
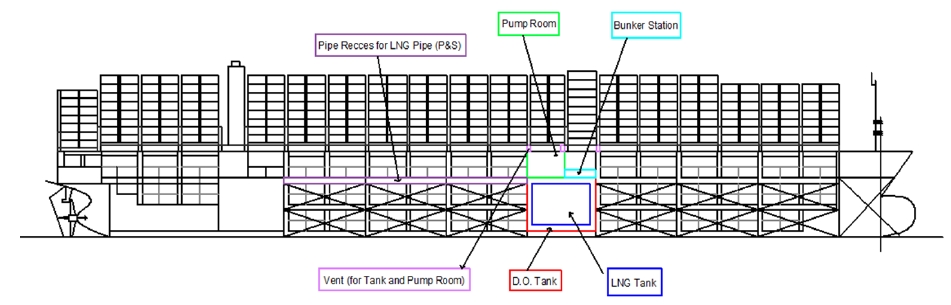
General General arrangement showing locations of bunker station, pump room, LNG tank, and pipe recess
The bunker station is located under the accommodation space on each side of the ship in a semi-enclosed space allowing the vessel to berth and bunker at any side. The bunker station has manifolds for liquid gas, vapor gas, nitrogen, and marine diesel oil.
The LNG is stored in a prismatic low-pressure insulated tank (A-type). Forced and natural boil off gas (BOG) is supplied to the main and auxiliary engines. The LNG tank is safeguarded by the B/5 location to the sides according to the IMO Interim Guidelines, with additional protection by the double hull, diesel oil tanks, including the structure of LNG tank itself hindering a potential penetration [8]-[10]. However, when sufficient impact energy overcomes the structural resistance of the outer hull, internal stringers, and bulkheads, the LNG tank may become punctured. Such high energy collisions are rare events and to date no collision resulting in the loss of cargo on LNG carriers has occurred [42].
The pump room is located between the upper deck and the “room for LNG tank”. This space is utilized for pumps, heaters, vaporizers, gas heaters, and compressors for the gas supply system to the main engine and auxiliary engines. The airlock space provides access from the underdeck passage.
Two pipe recesses for the gas supply system are provided below the underdeck passage on each side of the vessel, between the engine room and pump room. A single-wall pipe is arranged in each pipe recess. One pipe recess is arranged for the pipe for main engine, and the other for the pipe for the generator engines. The pipe duct for diesel oil pipes, water ballast pipes etc. is provided in the double bottom at the center of the ship.
The high-pressure gas supply piping is led to the engine room from the starboard side pipe recess, and the low-pressure gas supply piping is led to engine room from the port side pipe recess. The gas flow to the generator engines is regulated and measured in the gas valve unit (GVU) room located in the engine room. The gas to the main engine is regulated using the high-pressure (HP) pump.
The engine room arrangement is based on that of conventional container ships. However, the main engine type changed from a conventional two-stroke diesel engine to a dualfuel engine. The generator engine type is changed from a conventional four-stroke diesel engine to a dual-fuel engine. In addition, a high-pressure gas supply system for the main engine, and a low-pressure gas supply system for the generator engines are provided.
The boil-off gas from the LNG tank is burnt in the ship’s main propulsion engine and generators engines. Under normal operating conditions when the ship is at sea, one FG compressor, gas heater, LP pump, and HP pump operate to supply gas fuel to the ship’s main propulsion engine and generator engines. The FG compressor then discharges the gas to the engines via the gas heater. The HP vaporizer is used to discharge high-pressure gas to the main propulsion engine. If the fuel consumption of the main propulsion engine and generator engines cannot be met by the gas supplied by natural boil-off from the tank, additional gas is obtained by utilizing the HP vaporizer via the LP and HP pumps, where the vaporizer is fed by the liquid supply of these pumps. The outlet gas from the vaporizer is controlled by the gas heater.
3. Analysis Basis and Methodology
HAZID is a structured approach and involves exercises where documentation/drawings and a set of guidewords form the basis for identifying hazards involved with an operation or the use of equipment and/or systems. HAZIDs are commonly used throughout the maritime industry for all types of safety and risk assessments [12]-[17][45][47]-[52].
Figure 3 shows the vessel lifecycle. The focus of the HAZID assessment is the LNG fuel system and gas engines encompassing the following sequence of operations during the vessel’s lifecycle:
1. Construction/installation, including testing and sea trials.
2. Operations (loading/offloading of cargo, voyage, bunkering, docking, maintenance, lay-up/idle).
3. Decommissioning/scrapping
The safety of ship propulsion during a voyage and in maneuvering to avoid blackout has been taken into account.
The following hazard guidewords are used as a basis for the HAZID study [12]-[17][44][46]: fire or explosion hazard; fire/explosion – LNG initiated; fire/explosion – not LNG initiated, other hazards generated by materials and substances, leakage of liquid LNG causing loss of structural integrity, mechanical hazards, electrical hazards, thermal hazards, hazards generated by malfunctions, collisions, dropped object, grounding, foundering, environmental hazards, pollution, occupational accidents, hazards generated by neglecting ergonomic principles, and hazards generated by erroneous human intervention.
For each hazard cause/treatment/initiating event, consequences and controls (preventive and mitigating) are identified and presented in Section 4 (i.e., HAZID findings and results), following the Bow Tie hazard and effect model shown in Figure 4.
The diagram/model in Figure 4 is shaped like bowties, creating a clear differentiation between proactive and reactive hazards and effects. The hazard and top event always appear together in the center of the bow-tie diagram.
A hazard is a situation or object with the potential to cause harm. If the hazard is kept under control, then it is “safe” and unwanted consequences do not arise. A cause is an object or phenomenon that initiates a sequence of events that, if unchecked, leads to the top event. If a cause is present, and there are no barriers in place to intercept it, then the top event occurs. For example, over-pressurization could be a cause of loss of containment/tank of a hydrocarbon-carrying LNG/gas fuel. Causes appear on the left-hand side of the bow-tie diagram. Causes should be independent of each other and should lead to the top event directly. Causes should not be failures of equipment, as this is a barrier failure.
A consequence is an unwanted, undesirable, and potentially dangerous outcome of the top event occurring. A consequence results in loss or damage. It is common to think of consequences as affecting people, the environment, assets, business, and reputation. More safeguards/barriers are put in place to attempt to stop the top event from developing into consequences. Consequences appear on the right-hand side of the bow-tie diagram. Barriers control the top event, by either preventing its occurrence or preventing the consequences should it occur. Preventive barriers (safeguards) appear on the left of the diagram and are designed to prevent the top event from occurring. They should be seen to prevent each cause from resulting in the occurrence of the top event. Mitigation barriers appear on the right of the diagram. Given that control of the hazard is lost, they are designed to prevent the consequences. Barriers should only appear on one side of the bow-tie diagram, and not on both sides. Barriers can, however, appear on a number of cause lines simultaneously.
4. Findings and Results
The identified hazards are summarized in Table 2. Figure 5 classifies and ranks the main hazards and consequences. A total of 34 hazards were identified; this is ranked with respect to: fire/explosion – LNG initiated (19), loss of propulsion power (3), dropped objects (3), collision (2), fire/explosion - not LNG initiated (1), other hazards generated by materials and substances (1), leakage of liquid LNG causing loss of structural integrity (1), grounding (1), foundering (1), hazards during installation (1), and hazards during scrapping (1).
5. Recomendations
Eighteen recommendations with a comprehensive summary in terms of design and operation are made, covering a widerange of design and operation topics with the division in high prioritization for follow-up. Several important gaps beyond mandatory regulations, standards, and guidelines or of relevant organizations were identified requiring the following actions.
A. The following design recommendations should be considered for the safe operation of gas-fueled container ships based on Section 4 (i.e., HAZID findings and results):
(1) Thermal shielding of the ship structure using a water curtain.
A water curtain system covering the bunker area and side shell to mitigate damages in the case of LNG leakage should be considered. While the drip tray in the bunker station is intended for small leaks, the waterfall curtain will provide thermal shielding to protect and maintain the integrity of the ship’s structure, including tanks and neighboring equipment in case of larger liquid leaks during bunkering.
(2) Mechanical ventilation in bunker station.
Mechanical ventilation should be provided in the semi-enclosed bunker station to prevent any accumulation of gas. The bunker station is too enclosed to ensure efficient natural ventilation; thus, mechanical ventilation should be added.
(3) Avoiding blackout when automatic shutdown (ESD) is activated.
A gas leakage with required shutdown (ESD) functions should not damage the entire propulsion and power generation system, thus causing blackout. For system configurations with inherently safe machinery spaces, there are two situations where automatic shutdown of the gas supply to the engine room is required according to the international requirements for gas supply system safety functions. In addition, ESD should only be followed if there is gas detection in two detectors. Gas detection in one detector should sound an alarm.
(4) Full double-pipe arrangement for high-pressure piping.
Due to high pressure (300 bar) gas passing inside the pipe recess without double piping (only single pipe and ducting), full double pipe arrangement inside the pipe recess should be considered, considering crew safety and structural integrity.
However, protection of flanges may not be sufficient. Rupture of the pipe, rather than only leaking from connections, due to high pressure is possible. Alternatively, the pipe recess (ducting) should have sufficient constructive strength to maintain its structural integrity in the case of pipe rupture, e.g. pressure testing, and installed and protected so as to minimize the risk of injury to personnel in case of rupture.
(5) Airlocks for access to the GVU room.
Direct access through doors, gastight or otherwise, is generally not permitted from a gas-safe space to a gas-dangerous space, according to the IMO Interim Guidelines.
Non-hazardous spaces (engine room) with openings to hazardous areas (GVU room) should be arranged with an airlock and be maintained at an overpressure relative to the external hazardous area. This is to prevent any gas from the GVU room reaching the engine room, which contains non-EX rated equipment; this is because otherwise, it may ignite the gas.
(6) Redundant gas heating system for supply to generator engines.
Arrange for an additional heat exchanger for use as back-up for the one (1) gas heater currently proposed for the supply of gas to the generator engines. If the gas heater does not function, the vessel needs to vent the gas. This is not a safety critical issue, but an environmental concern.
(7) Locations of double-pipe ventilation air inlet and exhaust to be in safe positions. In addition, the locations of a vent mast is to be especially considered to prevent ignition from funnel and ingress into any air inlet. In addition, this ensures that vent piping is routed in a way that external leakages do not result in hazardous situations (e.g., release of gas to container spaces, accommodation).
(8) High-pressure components in the gas injection and system must be designed in accordance with international standards.
B. The following operational recommendations are considered for the safe operation of gas-fueled container ships based on Section 4 (i.e., HAZID findings and results):
(1) Attention to dropped objects, i.e., restrict/prohibit loading of containers near bunker station while bunkering.
Loading near the bunkering station is restricted/prohibited while bunkering LNG to avoid containers tipping over the side (on the water side/bunker shipside) or unintentionally being dropped on the bunkering hose or above the pump room. There is constant monitoring of the entire bunkering operation, and the use of guards. Company procedures are also established for special concerns regarding internal lifting activity in the pump room and protection of LNG equipment.
(2) Using checklist during bunkering.
Procedures/checklists are established between the ship owner and gas supplier for safe bunkering operation. The bunker station should have restricted access during bunkering operation, i.e., safety zone to be established. It must be ensured that the responsibilities during the LNG bunkering process are clearly defined for all foreseen LNG bunkering configurations and locations. The bunkering procedures are the preferred instrument with which to document the responsibilities during LNG bunkering.
(3) Entering hazardous areas, e.g., pump room and GVU room. Personal protective equipment is mandatory for entering the pump room owing to cold piping and high-pressure systems. In addition, training of personnel to operate the system is given.
(4) Harmonize the requirements for emergency repairs (including competence requirements of personnel performing these activities) of LNG-fueled vessels in shipyards, and develop initiatives to build competence and knowledge with regard to salvation of LNG -fueled vessels.
(5) The main potential for risk reduction is the potential for personnel to escape. Key aspects of this include ensuring that the detection of releases is communicated to personnel onboard effectively. In terms of minimizing risks to personnel, and from delayed ignition events in particular, the most effective mitigation is to escape to a place of safety, which is either to accommodation or suitable shelter or to an area outside the flammable cloud envelope. The former is the most reliable, although the latter is likely to be practicable in most cases (noting, however, that larger releases, e.g., due to collision or large leak during bunkering, cover a significant proportion of the vessel). Training is fundamental in escape, although measures such as temporary shelters or ensuring that accommodation can be used as required are recommended.
(6) Fatalities during evacuation by lifeboat or life raft, e.g., due to malfunctioning of the evacuation means, or as a consequence of the attempted escape by sea, may occur.
(7) Continuously promote the developments on the effect of methane number over dual-fuel engine operations. Operational guidelines need to be developed to reduce potential negative environmental impacts related to the possible release of methane. Establish a comprehensive approach for methane slip management, i.e., boil-off gas, vapor management, and emergency venting.
(8) It is further recommended to work actively to promote a strong and sound safety culture. Involvement by all parties in the organization in the process of defining, prioritizing, and controlling risk and hazards, along with a sense of shared purpose in safety, is important to the health and safety level onboard the vessel.
(9) The specific locations of Busan and Bandar Abbas ports for LNG Bunkering by ship-to-ship (STS) or other means must be separately assessed to identify HAZID (if any) and mitigate and eliminate potential hazards. The actual risk depends on the location of operation. Analysis of the following parameters, amongst others, is also taken into account:
- Operating environment (service conditions between various ships may differ, and these might result in different leak frequencies for otherwise similar equipment. Comparisons of data sets from different ships are difficult because of inconsistent reporting, varying standards of safety management, different types of fluid, and differences in environmental factors).
- Safety management (the quality of operation, inspection, maintenance etc. is a critical influence on leak frequencies; the leak frequencies for ships with lower standards may be higher).
- Materials (as different materials have different properties for corrosion, erosion, fatigue, etc., the materials used for the gas fuel system design are expected to affect the leak frequencies).
- Operating conditions of temperature/pressure (equipment operating close to its design pressure may be more vulnerable to accidental overpressure; also in addition, equipment operating above or below the normal temperature for its construction material may be more vulnerable to material failure).
- Equipment age (in theory, new equipment is vulnerable to teething problems and old equipment to wear-out, producing a bath-tub curve of failure rate versus time. Equipment that is subject to corrosion or fatigue is normally designed with a finite life, and the probability of failure increases as it nears the end of this period).
Process continuity (numerous failures occur during shutdown or start-up; failures are more likely in systems that experience many shutdowns).
- Manning levels (a high manning level is expected to increase the risk of process leaks as a large fraction of the registered leaks is related to human impact/intervention; thus, an increased activity level in the vicinity of the gas fuel system may increase the potential for damaging equipment).
(10) Arrangements for simultaneous bunkering and use of accommodation ladder near the bunker station are to be considered.
In summary, the proposed recommendations are taken into account and considered as follows:
(1) Work actively to promote a strong and sound safety culture. Involvement by all parties in the organization in the process of defining, prioritizing, and controlling risk and hazards, along with a sense of shared purpose in safety, is important to the health and safety level onboard the vessel.
(2) The bunkering procedures are the preferred instrument to document the responsibilities during LNG bunkering.
(3) The bunker station should have restricted access during bunkering operation, i.e., safety zone to be established.
(4) Personal protective equipment shall be mandatory for entering the pump room owing to cold piping and high-pressure systems.
(5) Training and competency of personnel to operate and maintain the system should be conducted and checked, respectively.
(6) Escape and evacuation routes and means are to be available continuously; at least two widely separated escape routes and two evacuation means must be present.
(7) Operational guidelines need to be developed to reduce potential negative environmental impacts related to the possible release of methane. Establish a comprehensive approach for methane slip management to mitigate and eliminate both environment and safety operation of engines.
(8) Parameters such as operating environment, safety management, materials specification/properties, operating conditions, process continuity, and manning levels for specific locations of LNG bunkering by STS or other means to be assessed to identify HAZID and mitigate and eliminate potential hazards (if any).
(9) A separate HAZID study and investigation shall be conducted on-site to select appropriate and feasible potential LNG bunkering methods and locations at Bandar Abbas port and Busan port considering the relevant hazards and risks at these locations. The focus of the selection bunkering methods (i.e., STS, truck-to-ship transfer via flexible hose, intermediate tank-to-ship transfer, and portable tank-to-ship bunkering methods) should also comply with relevant national regulatory requirements on usage of LNG as a marine fuel. The provision of bunkering services is to be considered part of the location selection and risk identification exercise.
Existing structures are to be evaluated in terms of capability of accommodating such activities without upgrading and rebuilding the jetty (technical feasibility and significant costs). The main selection criteria are the potential technical and operational capabilities of handling LNG bunkering in these areas, without requiring prohibitive infrastructure development. In addition, all the locations meet the requirement of being suitably distant from on-site operations and populations. The HAZID studies are aimed at answering the key question of whether LNG activities are possible at the proposed locations, from the perspective of major risks, public safety, and other activities in the direct vicinity. Where specific major risks or public safety issues are identified, the study advises on a set of possible mitigation measures.
The HAZID study for LNG bunkering at Bandar Abbas port and Busan port includes the following steps: assumption of terminal layout and fuel consumption of vessels; assessment of surrounding area of the location, including the potential presence of population, industrial areas, waterway traffic, and nautical layout; identification of major hazards and high-level assessments of credible events associated with the LNG bunkering operations; hazard identification study; identification of significant consequences that could imply strong arguments to effect the continuation of the present efforts on the proposed project and the necessary mitigation measures to enable the continuation of the project; risk ranking based on the consequence and likelihood.
The study should conclude that it is technically feasible to locate an LNG bunkering facility at the proposed locations/ jetties while meeting the requirements of local and international regulations and standards.
6. Conclusions
The overall and key results from the HAZID, covering the entire range of potential safety issues, various hazards that reached a level of safety and unsafe operation, and technical showstoppers, are identified. The HAZID results confirm that there are no major HSE showstoppers in terms of performing construction and conversion on dual-fueled vessels. The main selection criterion is the potential design, worst-case scenario for location of LNG tank below accommodation, and technical and operational capabilities in conducting the HAZID study and investigations.
The estimated HAZID increase is mainly due to the presence of the LNG tank and its effect on the risk from fire/explosions due to ship collision. A dropped container potentially penetrates the main deck and damages gas piping and equipment in the pump room if dropped from a great height. However, a dropped container only penetrates the main deck structure if no other containers are stored on the main deck. A dropped container also potentially damages/ruptures the bunker line/hose if dropped and tipped over the shipside and onto the bunker ship/barge, causing fire/explosion.
Several important gaps in mandatory regulations, standards, and guidelines or of relevant organizations are identified as requiring further action following the recommendations. However, this study concludes that it is technically feasible for the arrangement and installation of machinery for propulsion and auxiliary purposes, using natural gas as fuel, to have an equivalent level of integrity in terms of safety, reliability, and dependability as that of new and comparable conventional oil-fueled main and auxiliary machinery, while meeting the requirements of local and international regulations and standards.
The study and investigation concludes that there are no health, safety, and environment showstoppers for construction / conversion of conventional oil-fueled to dual-fueled LNG vessels for which HAZID can be mitigated and eliminated with sufficient design, engineering, and operational controls that meet the required standards.
References
- LNG Overview - US Federal Energy Regulatory Commission, www.ferc.gov/for-citizens/citizen-guides.asp. Accessed July 10, 2014.
- BSI, “Installations and equipment for liquefied natural gas. General characteristics of liquefied natural gas,” BS EN ISO 16903, June, 30), (2015.
- ISO, “Natural Gas – Calculation of calorific values, density, relative density and Wobbe index from composition,” ISO 6976:2016, Aug, 15), (2016.
- SIGTTO, Liquefied Gas Carriers – Your Personal Safety Guide: Witherby Seamanship International Ltd, 2nd Edition, (2012).
- ISO, “Natural Gas”, ISO 14532:2014, June, 15), (2014.
- MARPOL, International Convention for the Prevention of Pollution from Ships, (1983).
-
S. H. Han, Y. S. Yun, J. S. Kim, and Y. C. Lee, “A study on the development of educational programs for LNG bunkering in consideration of the safety system”, Journal of the Korean of Marine Environment & Safety, 22(3), p268-277, (2016).
[https://doi.org/10.7837/kosomes.2016.22.3.268]
- IGF Code, International Code for the Construction of Gas Fuelled Ships.
- The IMO Publication, “Interim guidelines on safety for natural gas fuelled engine installations in ships”, IMO Resolution MSC, 285(86), (2009).
- International Maritime organisation (IMO)-SOLAS, “The international convention for the safety of life at sea”, (2014).
- SIGTTO, Liquefied Gas Fire Hazard Management: Witherby Seamanship, (2004).
- Directive 99/92/EC – Risks from Explosive Atomospheres, 1999.
- Directive 94/9/EC (also known as ‘ATEX 95’ or ‘the ATEX Equipment Directive’) is on the approximation of the laws of Members States concerning equipment and protective systems intended for use in potentially explosive atmospheres.
- BSI, “Explosive atmospheres,” BS EN 60079, April, 30), (2009.
- BSI, “Explosive atmospheres gas detectors – Selection installation, use and maintenance of detectors for flammable gases and oxygen,” BS EN 60079-Part 29-2, (2007).
- SIGTTO, Contingency Planning and Crew Response Guide for Gas Carrier Damage at Sea and in Port Approaches: Witherby Seamanship, (1999).
- ISO, “Materials, equipment and offshore structures for petroleum, petrochemical and natural gas industries,” ISO/TC 67.
- 2014/94/EU of the European Parliament and of the Council, “Directive on deployment of alternative fuels infrastructure”, OCT, 22), (2014.
-
J. de Wit, “EEMUA recommendations for the design and construction of refrigerated liquefied gas storage tanks”, Cryogenics, 28(12), p800-804, (1988).
[https://doi.org/10.1016/0011-2275(88)90174-9]
- BSI, “Installation and equipment for liquefied natural gas – Design and testing of marine transfer systems – Part 1: Design and testing of transfer arm,” BS EN ISO 16903, June, 30), (2015.
- ISO, “Guidelines for systems and installations for supply of LNG as fuel to ships,” ISO/TS 18683, Jan, 15), (2015.
- ISO, “Liquefied natural gas installations and equipment,” ISO/TC 67/SC 9, (2015).
- SIGTTO, The selection and testing of valves for LNG applications, http://www.sigtto.org/media/7205/ selection-and-testing-of-valves-for-lng.pdf Accessed, (2008).
- ISO, “Guidelines for systems and installations for supply of LNG as fuel to ships,” ISO /TS 18683, Jan, 13), (2015.
- NMD, “Regulation of the Norwegian maritime directorate concerning the construction and operation of gas-fuelled passenger ships,” Regulation No. 1218, Sep, 09), (2005.
- SIGTTO, ESD Arrangements and Linked Ship/Shore Systems for Liquefied Gas Carriers, http://www.sigtto.org/media/7201/esd-arrangements-and-linked-ship-shore-systems.pdf (2009).
- ISO, “Petroleum and natural gas industries - Design and testing of LNG marine transfer arms for conventional onshore terminals,” ISO 16904, ISO/TC 67/SC 9, Feb, 발행12,일), (2016.
- BSI, “Installation and Equipment for LNG – Design and testing of marine transfer systems – Part 2: Design and testing of transfer hoses,” BS EN 1474-2, March, 13), (2009.
- SIGTTO, Manifold Recommendations for Liquefied Gas Carriers: Witherby Seamanship International Ltd, (2011).
- IEC TC/SC 18, “Electrical installations in ships: Tankers - Special features: IEC,” IEC 60092-502, (1999).
- BSI, “Specification for metallic hose assemblies for liquid petroleum gases and liquefied natural gases,” BS 4089, Dec, 15), (1999.
- NFPA, “Standard for the production, Handling and storage of liquefied natural gas (LNG),” NFPA 59A – 2016.
- BSI, “Installation and equipment for LNG - Design of onshore installations,” BS EN 1473, May, 31), (2016.
- Guidance on performing risk assessment in the design of onshore LNG installations including the ship/shore interface – ISO draft 116901, 2015.
- American Bureau of Shipping, Guide for Propulsion and Auxiliary Systems for Gas Fueled Ships, American Bureau of Shipping Publication, June, 20), (2015.
- SIGTTO, A Risk Based Approach for the Provision of Firefighting Equipment on Liquefied Gas Jetties: Witherby Seamanship, (1999).
- B. Veritas, “Safety rules for gas-fuelled engine installations in ships,” NR 529, May), (2011.
- S. Mokhatab, J. Y. Mak, J. V. Valappil, and D. A. Wood, LNG Fire Protection and Emergency Response, Institution of Chemical Engineers, Texas, USA, (2007).
- SIGTTO, Accident Prevention - The Use of Hoses and Hard-Arms at Marine Terminals Handling Liquefied Gas: Witherby Seamanship, Canada, (1996).
- SIGTTO, The Selection and Testing of Valves for LNG Applications, http://www.sigtto.org/media/7205/selection-and-testing-of-valves-for-lng.pdf Accessed January 15, 2016.
- BSI, “Installation and equipment for LNG design and testing of marine transfer systems - Offshore transfer systems,” BS EN 1474-3, June, 30), (2008.
- J. L. Woodward, and R. M. Pitblado, LNG Risk based Safety – Modelling and consequence analysis, Wiley, Hoboken, New Jersey, Canada, (2015).
-
C. J. Deetjen, R. Pitblado, and T. Hysing, “Energy-Based Methodology for Collision Protection for LNG Carriers”, Offshore Technology Conference, (2008).
[https://doi.org/10.4043/19516-ms]
- SIGTTO, Guidance for the Prevention of Rollover in LNG Ships, July), (2012.
- SIGTTO, ESD Arrangements & linked ship/shore systems for liquefied gas carriers, http://www.sigtto.org/media/7201/esd-arrangements-and-linked-ship-shore-systems.pdf Accessed January 15, 2016.
- IACS, Formal Safety Assessment of general Cargo Ships Preparatory step, http://www.iacs.org.uk/document/public/Publications/Other_technical/PDF/_FSA_General_Cargo_Ships_Preparatory_Step_pdf848.pdf Accessed September 15, 2008.
- OGP, Risk Assessment Data Directory; Vulnerability of humans, Report No. 434-14-1, International Association of Oil and Gas Producers, (2010).
- SAFEDOR WP 4.2 FSA of RoPax ships, Design and Operation and Regulation for Safety, 2012.
-
M. Hightower, L. Gritzo, A. Luketa-Hanlin, J. Covan, S. Tieszen, G. Wellman, M. Irwin, M. Kaneshige, B. Melof, C. Morrow, and D. Ragland, “Guidance on risk analysis and safety implications of a large liquefied natural gas (LNG) spill over water”, SAND2004-6258, Sandia Report United States, (2004).
[https://doi.org/10.2172/882343]
- J. L. Woodward, and R. M. Pitblado, LNG Risk Based Safety, Modelling and Consequence Analysis, Wiley, Canada, (2010).
- J. G., “Quintiere, smoke measurements: An assessment of correlations between laboratory and full-scale experiments”, Fire and Materials, 6(3-4), p145-160, (1982).
- NTS, “Risk and emergency preparedness analysis,” Rev 2, NORSOK Standard Z-013, (2001).
- ISO, “Risk mangaement – Risk assessment techniques,” IEC/ ISO 31010, ISO/TC 262, Dec, 01), (2009.