
Optimizing fuel injection timing: Investigating the effects of injection timing on combustion performance and emission characteristics of high and low sulfur fuels in a diesel engine
Copyright © The Korean Society of Marine Engineering
This is an Open Access article distributed under the terms of the Creative Commons Attribution Non-Commercial License (http://creativecommons.org/licenses/by-nc/3.0), which permits unrestricted non-commercial use, distribution, and reproduction in any medium, provided the original work is properly cited.
Abstract
Owing to environmental considerations, there is an increased interest in the Maritime Industry in regulating sulfur oxide emissions and maintaining engine performance. This necessitates installing exhaust gas cleaning systems onboard ships that are costly or use alternative fuels and low-sulfur marine fuels under 0.50%, as set by the International Maritime Organization. In addition, injection timing optimization greatly influences the performance and emission characteristics of diesel engines. Hence, this study investigates the effects of combining high-sulfur, low-sulfur, and ultra-low-sulfur fuels with fuel injection timing using a 3D simulation model from AVL FIRE R2022a while maintaining constant initial and boundary conditions. The results show that the use of light marine diesel fuel oil and Diesel-EN590 causes an increase in the in-cylinder temperature and pressure compared to heavy fuel oil. Moreover, the results show a significant decrease in emissions, especially soot emissions, and a slight decrease in NOx and CO2 emissions. Advanced injection timing results in increased peak pressure and in-cylinder temperature. Considering the emission characteristics, the optimal injection timing of 15CAD promotes CO2 reduction, whereas 10CAD promotes NOx emission reduction, although slight variations existed. Therefore, this research strategy effectively improves the engine performance and reduces emissions, thereby mitigating energy and environmental crises.
Keywords:
High-sulfur fuel oil, Low-sulfur fuel oil, Ultra-low-sulfur fuel oil, Injection timing, Combustion performance, Emission1. Introduction
The rapid growth of the maritime industry has resulted in a significant increase in the use of fossil-fueled propulsion systems. Over 80% of global trade is carried out through international shipping, amounting to a daily fuel consumption of over 640,000 tons and 49.5% of the world’s residual fuel oil demand [1]. The increased fuel consumption in the marine industry has led to an energy crisis and increased emissions, resulting in the demand for higher fuel efficiency. Ship emissions include nitrogen oxides (NOx), sulfur oxides (SOx), carbon dioxide (CO2), carbon monoxide (CO), and particulate matter (PM). These emissions contribute to greenhouse effects; serious health problems such as premature deaths; cardiovascular, respiratory, and pulmonary diseases; and an increase in sea levels [2]-[4]. Thus, significant challenges exist for the marine engine sector in terms of reducing ship emissions while maintaining engine performance.
To reduce ship emissions, the International Maritime Organization (IMO) has introduced stricter emission regulations, including a Sulfur Cap for SOx, Tier III for NOx, and the implementation of a greenhouse gas strategy [5][6]. The global sulfur cap, which went into effect on January 1, 2020, aimed to reduce the sulfur limit of fuels from 3.50% to 0.50% for ships operating outside designated emission control areas and imposed a stricter limit of 0.10% in emission control areas (ECAs). The emission regulations have presented a great challenge in the shipping industry, and the three best strategies highlighted to comply with the IMO sulfur regulations include the use of fuels with lower amounts of sulfur; installation of exhaust gas cleaning systems (EGCS), including scrubbers; and use of alternative fuels, such as liquefied natural gas, alcohol-based fuels, ammonia, and hydrogen [7].
The use of EGCS, which can either be wet or dry scrubbers, to eliminate SOx from both engine and boiler exhaust gases has several advantages, including a reduction in operational costs by using cheaper, high-sulfur fuel. Moreover, the byproducts of dry scrubbers may be recycled and used to produce cement or fertilizers. However, this option incurs high installation and time costs resulting from ship downtime. In addition, the crew workload increases during the operation of the EGCS due to emission monitoring services and other areas have legal restrictions regarding the use of EGCS [8]. The other option of using low-sulfur fuel oils, which are products of refined oils, does not require the use of EGCS and can be used in heavy fuel oil (HFO) blends. In comparison, the alternative of using either marine fuels with lower amounts of sulfur, per the required limits, or other alternative fuels offers a suitable solution for the shipping industry to resolve future energy and environmental crises [9].
Several studies have proved that the use of conventional fuels with low sulfur contents, such as marine gas oil (MGO) and light marine diesel oil (MDO) with sulfur contents ranging from 0.1% to 1.0%, very-low-sulfur fuel oil (VLSFO) with a sulfur content of less than 0.5%, ultra-low sulfur (ULSFO) with a sulfur content equal to or less than 0.1%, and fuel blends of MGO and ULSFO with HFO, reduces not only sulfur oxides but also other pollutants [10][11]. However, certain considerations arise when employing treated or blended VLSFO and ULSFO in diesel engines, such as an increased pour point, reduced lubricity, and incompatibility.
In addition to the use of low-sulfur fuel oils, the continuous improvement of diesel engine combustion performance and reduction of emissions are linked to fuel injection system optimization. Fuel injection timing, injection pressure, and injection duration are the primary factors that significantly affect engine performance and emission characteristics [12][13]. Several studies have demonstrated that advanced injection timing enhances an engine’s combustion performance, extends the ignition delay, and improves the air-fuel blend in the cylinders [14][15]. In addition, engine exhaust emissions can be significantly reduced via appropriate injection timing, which has great significance in solving environmental issues [16][17].
Therefore, the purpose of this study is to optimize diesel engine performance and reduce exhaust emissions by combining low-sulfur fuel oils with different fuel injection timing strategies using the AVL FIRE R2022a simulation software, aiming to contribute to resolving environmental and energy crises.
2. Methodology
2.1 Experimental Setup
In this study, a DOOSAN P158LE-111 engine running on an MDO with a low sulfur content was used to analyze the effects of injection timing on combustion performance and emission characteristics. Table 1 lists the specifications of the main engine.
Table 2 lists the properties of the MDO used in the experimental analyses.
2.2 Three-Dimensional Simulation Models of the In-Cylinder Flow Combustion
In this analysis, three-dimensional (3D) simulations were conducted from the inlet valve closing (IVC) to exhaust valve opening (EVO) using the AVL FIRE R2022a simulation software. Highly advanced models in the AVL FIRE simulation software are appropriate for accurately simulating the combustion and emission characteristics of diesel, gasoline, and dual-fuel engines [18]-[21].
The generation of a computational mesh of the combustion chamber is essential when modeling the simulation. Owing to the axial symmetry of the engine and the presence of a fuel nozzle with ten identical holes, one-tenth of the entire 3D computational mesh of the combustion chamber was modeled to reduce the computation time. Figure 1 shows the two-dimensional (2D) and 3D sector computational meshes as well as the 2D sketch of the piston shape, injector position, and direction of injection.
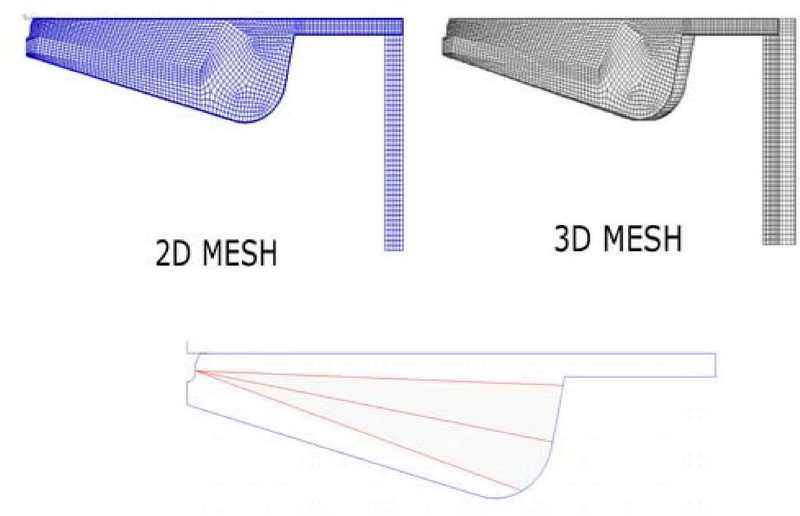
2D and 3D sector computational meshes and 2D sketch of piston shape, injection nozzle position, and direction of injection
The engine operational boundary and initial condition parameters, which play critical roles in obtaining accurate, stable, and reliable results, were obtained from the experimental analysis to enhance the design optimization of the simulation model. Tables 3 and 4 show the boundary and initial conditions, respectively.
Various models, including turbulence, combustion, ignition, atomization, and emission (NOx and soot) models have been used to accurately perform engine simulations using AVL FIRE R2022a. The turbulence model used in the simulation is the k-ζ-f model, which is an improved four-equation turbulence model based on the simple k-ε model that provides higher accuracy and stability turbulence prediction [22].
A 3-zones extended coherent flame species transport model was employed to study the combustion process. This model is outstanding compared with other coherent flame models, because of its partitioning of the combustion zone features into pre-flame, flame, and post-flame zones, which thereby increases the overall accuracy of the results [23].
Due to its high accuracy over a wide range of equivalent ratios, the extended Zeldovich model was employed to simulate the NOx emissions. These emission form in the engine combustion chamber when atmospheric nitrogen in the charge air reacts with atmospheric oxygen at high temperatures during the combustion process [24].
A kinetic soot mechanism model was used to model the formation of soot as a result of the incomplete combustion in internal combustion engines. By precisely calculating and predicting all chemical reactions, particle development, and particle combustion steps, this model can effectively represent the amount and characteristics of emissions, depending on the engine operating conditions [24].
An autoignition model was employed for ignition. Moreover, the WAVE, Dukowicz, and Walljet1 models were utilized in the atomization process, which involves breaking liquid fuel into smaller droplets, evaporation, and droplet wall interaction processes [25]. Table 5 lists these models.
2.3 Simulation Case Description
A comparative analysis was conducted to investigate the impacts of various levels of sulfur content in fuels and the effects of injection timing on diesel engine combustion, that is, the in-cylinder pressure, in-cylinder temperature, brake-specific fuel consumption, and emission characteristics for NOx, CO2, and soot. To investigate the effects of various levels of sulfur content in fuels and injection timing, simulation models of HFO with high sulfur content, MDO with low sulfur content, and Diesel EN590 (D-EN590) with ultra-low sulfur content were modeled. Table 6 lists the fuel properties. Simultaneously, to investigate the effects of the injection timing, the variations in the injection timing from advanced to late ignition timing included 15CAD BTDC, 10CAD BTDC, and 5CAD BTDC. During the simulation, the total amount of fuel injected into the engine and all other boundary, initial conditions, and engine operating conditions remained constant.
2.4 Simulation Model Validation
The MDO simulation results were compared with the experimental results to verify the reliability and accuracy of the simulation model. The experimental values used for the validation were the brake mean effective pressure, indicated specific power, and carbon dioxide (CO2) mass fractions. The deviations in the brake mean effective pressure, indicated specific power, and CO2 mass fraction were 4.91%, 0.78%, and 4.68%, respectively. Figure 2 shows a comparison of the experimental and simulation results.
2.5 Mesh Independence Analysis
Mesh independence analysis is an important approach for investigating whether the simulation results are independent of the geometric mesh size. Therefore, to establish the accuracy of the results and keep the computational costs low, combustion simulations were conducted on three developed meshes, that is coarse, medium, and fine, at the standard injection timing of 16CAD BTDC. Table 7 lists the mesh properties and calculation times.
Figure 3 shows the results of the in-cylinder temperature and pressure obtained when three mesh resolutions were used. The final results were independent of the three meshes used in this study. Therefore, the use of any of the three mesh configurations yielded accurate results. Mesh 2 (medium) was selected for use in the simulations for the entire study because of its reasonable calculation time and the accurate results obtained during the mesh independence study.
3. Simulation Results and Discussions
3.1 In-Cylinder Peak Pressure
Figure 4 shows the variations in the in-cylinder pressures of different fuels at different injection timings, using the same total amount of fuel injected. The in-cylinder pressure profiles of all fuels were similar, although there were small pressure variations between fuels. For example, at SOI 15CAD BTDC, D-EN590 exhibited the highest pressure of 204.23 bar, followed by MDO and HFO with 203.82 bar and 202.70 bar, respectively. This variation is attributable to the differences in viscosity, cetane number, and calorific value. The lower the cetane number in a fuel, the slower the combustion rate; hence, the cylinder pressures are slightly lower than those of other fuels. In addition, HFO has a lower calorific value than do MDO and D-EN590, which results in the release of less energy per unit time during combustion and thus lowers the overall in-cylinder pressure [26]. In terms of injection timing, the in-cylinder pressure increases with the advancement of injection timing. The in-cylinder pressure was highest at 15CAD, and this was followed by 10CAD and 5CAD BTDC. These results are due to the longer ignition delay, which results in more fuel and air blends, and thus more efficient combustion [27].
3.2 In-cylinder mean temperature
Figure 5 shows the variations in the in-cylinder mean temperatures of the different fuels at different injection timings under the same fuel injection conditions. The in-cylinder temperatures of the various fuels exhibit small variations. At 15CAD BTDC, D-EN590, MDO, and HFO exhibit temperatures of 1899.82 K, 1896.68 K, and 1895.63 K, respectively. These results are attributed to the differences in the cetane numbers and atomization efficiencies of the fuels, which result from the differences in their viscosities. Specifically, lower viscosity leads to more efficient and complete combustion, even though the calorific values of the three fuels are in the same range. The advancement of the injection timing resulted in increased in-cylinder temperatures, owing to the increased fuel-air blended mixture in the combustion chamber [28]. However, the in-cylinder temperatures were slightly higher at 5CAD BTDC than at 10CAD BTDC, due to the shorter ignition delays at peak pressures and high fuel temperatures [29].
3.3 Nitrogen Oxide (NO) Emissions
Figure 6 illustrates the NO mass fractions of different fuels at different injection timing for the same amount of injected fuel. Temperatures above 1800 K, oxygen content, and reaction time inside the engine cylinder had the most significant effects on NO production. HFO has a higher brake fuel oil consumption of 0.266 kg/kWh than do MDO and D-EN590, which have consumptions of 0.255 kg/kWh and 0.252 kg/kWh, respectively. Thus, the peak in-cylinder temperature reaction with atmospheric nitrogen in the combustion chamber combined with fuel NO produced, owing to the high nitrogen content in HFO, results in the ideal formation of higher NOx in HFO, compared with MDO and D-EN590 [30]. In terms of injection timing, which has an influence on the ignition delay time and ignition energy produced where the temperature curves are highest, that is, 5CAD BTDC, NOx formation increased [31].
3.4 Carbon Dioxide (CO2) Emissions
Figure 7 shows the CO2 mass fractions of different fuels at different injection timings using the same amount of total fuel injected. CO2 emissions from the combustion chamber depend on several factors, such as the amount of carbon atoms in the fuel, amount of fuel injected, and combustion quality. CO2 is formed when the produced CO is further oxidized, owing to the presence of a sufficient concentration of oxygen and in-cylinder temperature conditions [32]. HFO produced slightly higher carbon dioxide emissions, compared with MDO and D-EN590. These results are attributable to the high carbon content of HFO and its poor combustion efficiency [33]. Furthermore, the injection timing at the top dead center is accompanied by high temperatures, which leads to high CO2 formation, as high temperatures facilitate the oxidation of CO to CO2 [34].
3.5 Soot Emissions
Figure 8 shows the soot mass fractions of different fuels at different injection timings for the same amount of injected fuel. The soot emissions from HFO were significantly higher than those from MDO and D-EN590. These results are attributable to the higher viscosity and poor volatility of the fuel, which led to poor fuel atomization and incomplete combustion [35]. In addition, the soot mass fractions were lower at injection timings with higher in-cylinder temperatures. The D-EN590 values at 15CAD, 10CAD and 5CAD BTDC were 0.040%, 0.039%, and 0.025%, respectively, because high temperatures enhance soot oxidation and thereby reduce the soot mass fraction [36].
3.6 HFO, MDO, and D-EN590
The use of various types of sulfur fuel oils, such as HFO, MDO, and D-EN590, has been shown to cause significant changes in the in-cylinder combustion process. These changes are mainly attributable to the properties of the fuels, including the density, sulfur content, and chemical composition. The density of HFO is comparatively higher than those of MDO and D-EN590 and therefore result in slow combustion rates and lower in-cylinder temperatures and pressures than those of the other two fuels, which leads to high soot mass fractions, as shown in the Figure 8. In addition, the high viscosity of HFO, compared with those of MDO and D-EN590, requires proper heating; otherwise, high viscosity leads to poor atomization, which deteriorates the combustion process, reduces the thermal efficiency of the engine, and compromises exhaust gas emissions. In conclusion, the lower sulfur content in both the MDO and D-EN590 fuels results in the lower production of SOx, enhanced cleaner combustion, and alleviation of environmental crises. Therefore, the above analysis proves that the need to switch to low-sulfur fuels impacts not only SOx emissions but also other in-cylinder combustion performance characteristics and exhaust gas emissions.
4. Conclusion
This study investigated the combined effects of fuel injection timing and the use of various types of sulfur fuel oils from HFO, MDO, and D-EN590, which is an ultra-low-sulfur fuel oil, in enhancing the combustion performance and reducing the emission characteristics of diesel engines. The main conclusions are as follows.
- 1) The use of D-EN590 led to a slight increase in the in-cylinder pressure and temperature, which promoted enhanced combustion efficiency, compared with MDO and HFO.
- 2) The use of D-EN590 resulted in a greater decrease in soot emissions than in NOx and CO2 emissions. Compared with HFO, D-EN590 significantly reduced exhaust emissions. However, a few insignificant differences were observed between the MDO and D-EN590 groups.
- 3) Early fuel injection timing at 15CAD and 10CAD BTDC led to an increase in the ignition delay, and hence an increase in the percentage of premixed combustion, resulting in a higher peak in-cylinder pressure and temperature. Therefore, the optimal SOI based on in-cylinder pressure and temperature is between 15CAD and 10CAD BTDC.
- 4) Furthermore, for D-EN590 at 15CAD and 10CAD BTDC, the NO emissions are lower by 0.044% and 0.043%, respectively, whereas the NO emissions are higher by 0.055% at 5 CAD BTDC. These results indicate that 10CAD is the optimal SOI.
- 5) The 15CAD BTDC SOI of D-EN590 had the lowest CO2 emissions of 12.70%, compared with 10CAD and 5CAD BTDC, which showed emissions of 13.43% and 14.28%, respectively. These results indicate that 15CAD is the optimal SOI.
- 6) The 5CAD BTDC showed a slightly high soot reduction, but considering other combustion characteristics, such as pressure and temperature, it does not offer an optimal SOI.
Therefore, based on the aforementioned conclusions on combustion characteristics and emissions, the need to switch to low-sulfur content fuels and effective fuel injection timing between 15CAD and 10CAD BTDC is essential for enhancing diesel engine combustion performance and reducing emissions.
Acknowledgments
This research was supported by the Technology Innovation Program (RS-2024-00459805, development of fuel supply equipment for methanol fueled ship) funded by the Ministry of Trade, Industry & Energy (MOTIE, Korea); and the ‘Technology development Program [S3366238]’ funded by the Ministry of SMEs and Startups (MSS, Korea).
Author Contributions
Conceptualization, Methodology, Software, Validation, Formal Analysis, Visualization, Writing-Original Draft Preparation, Writing-Review & Editing, N. I. Ahmed; Formal Analysis, Final review, J. K. Kim; Conceptualization, Formal analysis, K. W. Chun; Methodology, Project Administration, J. H. Choi; Conceptualization, Supervision, Project Administration, Writing-Review & Editing, W. J. Lee.
References
-
S. Vedachalam, N. Baquerizo, and A. K. Dalai, “Review on impacts of low sulfur regulations on marine fuels and compliance options,” Fuel, vol. 310, 122243, 2022.
[https://doi.org/10.1016/j.fuel.2021.122243]
-
L. Huang, Y. Wen, X. Geng, C. Zhou, and C. Xiao, “Integrating multi-source maritime information to estimate ship exhaust emissions under wind, wave and current conditions,” Transportation Research Part D: Transport Environment, vol. 59, pp. 148-159, 2018.
[https://doi.org/10.1016/j.trd.2017.12.012]
-
L. Bilgili, “Life cycle comparison of marine fuels for IMO 2020 Sulphur Cap,” Science of the Total Environment, vol. 774, 145719, 2021.
[https://doi.org/10.1016/j.scitotenv.2021.145719]
-
M. K. Kang, K. Y. Min, S. U. Park and J. D. Choi, “An experimental study on the air pollutant emission characteristics of high-speed diesel engine for small coastal ships according to the application of the LP EGR and DOC-DPF systems,” Journal of Advanced Marine Engineering and Technology, vol. 46, no. 1, pp.1-8, 2022.
[https://doi.org/10.5916/jamet.2022.46.1.1]
- International Maritime Organization (IMO), https://www.imo.org/en/OurWork/Environment/Pages/2023-IMO-Strategy-on-Reduction-of-GHG-Emissions-from-Ships.aspx, , Accessed December 7, 2023.
-
M. A. Je, S. H. Jung, T. Jeong, S. Hwang, and J. S. Moon, “Exhaust Gas Recirculation (EGR) effect on two-stroke diesel engines under sailing condition,” Journal of Advanced Marine Engineering and Technology, vol. 48, no. 1, pp.1-8, 2024.
[https://doi.org/10.5916/jamet.2024.48.1.1]
-
V. D. Tran, A. T. Le, and A. T. Hoang, “An experimental study on the performance characteristics of a diesel engine fueled with ULSD-biodiesel blends,” International Journal of Renewable Energy Development, vol. 10, p. 183, 2021.
[https://doi.org/10.14710/ijred.2021.34022]
-
V. V. Komissarova and E. V. Krasova, “The Scrubbers on the marine transport vessels: Technical, economic and environmental characteristics,” In 2020 International Multi-Conference on Industrial Engineering and Modern Technologies (FarEastCon), pp. 1-7, 2020.
[https://doi.org/10.1109/FarEastCon50210.2020.9271466]
-
S. Kumar and G. Goga, “Emission characteristics & performance analysis of a diesel engine fuelled with various alternative fuels–a review,” Materials Today: Proceedings, 2023.
[https://doi.org/10.1016/j.matpr.2023.02.457]
-
Z. Korczewski, “Research on the effect of low-sulphur marine fuels on the dynamic characteristics of a CI engine,” Combustion Engines, vol. 194, no. 3, pp. 97-103, 2023.
[https://doi.org/10.19206/CE-168390]
-
T. Chu-Van, N. Surawski, Z. Ristovski, C. -S. Yuan, S. Stevanovic, S. A. Rahman, F. M. Hossain, Y. Guo, T. Rainey, and R. J. Brown, “The effect of diesel fuel sulphur and vanadium on engine performance and emissions,” Fuel, vol. 261, 116437, 2020.
[https://doi.org/10.1016/j.fuel.2019.116437]
-
C. Cengiz and S. O. Unverdi, “A CFD study on the effects of injection timing and spray inclusion angle on performance and emission characteristics of a DI diesel engine operating in diffusion-controlled and PCCI modes of combustion,” Energies, vol 16, no.6, 2861, 2023.
[https://doi.org/10.3390/en16062861]
-
S. A. Rahman, H. Masjuki, M. Kalam, A. Sanjid, and M. Abedin, “Assessment of emission and performance of compression ignition engine with varying injection timing,” Renewable and Sustainable Energy Reviews, vol. 35, pp. 221-230, 2014.
[https://doi.org/10.1016/j.rser.2014.03.049]
-
B. Jayashankara and V. Ganesan, “Effect of fuel injection timing and intake pressure on the performance of a DI diesel engine–A parametric study using CFD,” Energy Conversion and Management, vol. 51, pp. 1835-1848, 2010.
[https://doi.org/10.1016/j.enconman.2009.11.006]
-
H. J. Kim, S. H. Park and C. S. Lee, “Impact of fuel spray angles and injection timing on the combustion and emission characteristics of a high-speed diesel engine,” Energy, vol. 107, pp. 572-579, 2016.
[https://doi.org/10.1016/j.energy.2016.04.035]
-
J. Liu, A. Yao, and C. Yao, “Effects of injection timing on performance and emissions of a HD diesel engine with DMCC,” Fuel, vol. 134, pp. 107-113, 2014.
[https://doi.org/10.1016/j.fuel.2014.05.075]
-
G. A. P. Rao and S. Kaleemuddin, “Development of variable timing fuel injection cam for effective abatement of diesel engine emissions,” Applied Energy, vol. 88, pp. 2653-2662, 2011.
[https://doi.org/10.1016/j.apenergy.2011.02.011]
-
V. C. Pham, V. V. Le, S. Yeo, J. -H. Choi, and W. -J. Lee, “Effects of the injector spray angle on combustion and emissions of a 4-stroke natural gas-diesel DF marine engine,” Applied Sciences, vol. 12, 11886, 2022.
[https://doi.org/10.3390/app122311886]
-
D. T. Nguyen, M. T. Vu, V. V. Le, and V. C. Pham, “Impacts of charge air parameters on combustion and emission characteristics of a diesel marine engine,” Thermo, vol. 3, no. 3, pp. 494-514, 2023.
[https://doi.org/10.3390/thermo3030030]
-
G. Bellér, I. Arpad, J. Kiss, and D. Kocsis, “AVL Boost: a powerful tool for research and education,” In Journal of Physics: Conference Series, vol. 1935, 012015, 2021.
[https://doi.org/10.1088/1742-6596/1935/1/012015]
-
S. Wei, K. Ji, X. Leng, F. Wang, and X. Liu, “Numerical simulation on effects of spray angle in a swirl chamber combustion system of DI (direct injection) diesel engines,” Energy, 75, pp. 289-294, 2014.
[https://doi.org/10.1016/j.energy.2014.07.076]
-
J. K. Kim, S. Yeo, J. -H. Choi, and W. -J. Lee. “LPG, gasoline, and diesel engines for small marine vessels: A comparative analysis of eco-friendliness and economic feasibility,” Energies, vol. 17, p. 450, 2024.
[https://doi.org/10.3390/en17020450]
- AVL FIRE®2018a. Combustion Module User Manual; AVL List GmbH: Graz, Austria, 2018.
- AVL FIRE®2018a. Emission Module User Manual; AVL List GmbH: Graz, Austria, 2018
- AVL FIRE®2018a. Spray Module User Manual; AVL List GmbH: Graz, Austria, 2018.
- T. Chountalas and M. Founti, “Effect of low-sulfur fuel on auxiliary engine combustion and performance,” Proceedings of the SNAME International Symposium on Ship Operations, Management and Economics, p. D021S003R002, 2021.
-
J. Li, Z. Zhang, Y. Ye, W. Li, T. Yuan, H. Wang, Y. Li, D. Tan, and C. Zhang, “Effects of different injection timing on the performance, combustion and emission characteristics of diesel/ethanol/n-butanol blended diesel engine based on multi-objective optimization theory,” Energy, vol. 260, 125056, 2022.
[https://doi.org/10.1016/j.energy.2022.125056]
-
H. G. How, H. H. Masjuki, M. A. Kalam, and Y. H. Teoh. “Influence of injection timing and split injection strategies on performance, emissions, and combustion characteristics of diesel engine fueled with biodiesel blended fuels,” Fuel, vol. 213, pp. 106-114, 2018.
[https://doi.org/10.1016/j.fuel.2017.10.102]
-
J. C. Ge, J. Y. Kim, B. O. Yoo, and J. H. Song, “Effects of engine load and ternary mixture on combustion and emissions from a diesel engine using later injection timing,” Sustainability, vol. 15, 1391, 2023.
[https://doi.org/10.3390/su15021391]
-
A. I. Tariq and A. Saleh, “An experimental investigation into the combustion properties, performance, emissions, and cost reduction of using heavy and light fuel oils,” Case Studies in Thermal Engineering, vol. 44, 102832, 2023.
[https://doi.org/10.1016/j.csite.2023.102832]
-
A. Alghafis and E. A. Raouf, “Optimization of injection timing and injection duration of a diesel engine running on pure biodiesel SME (Soya Methyl Ester),” Open Journal of Applied Sciences, vol. 10, p. 486, 2020.
[https://doi.org/10.4236/ojapps.2020.107034]
-
A. J. Nyongesa, M. H. Park, C. M. Lee, J. H. Choi, V. C. Pham, J. J. Hur, and W.J. Lee. “Experimental evaluation of the significance of scheduled turbocharger reconditioning on marine diesel engine efficiency and exhaust gas emissions,” Ain Shams Engineering Journal, vol. 15, no. 8, 102845, 2024.
[https://doi.org/10.1016/j.asej.2024.102845]
-
W. Ramsay, E. Fridell, and M. Michan, “Maritime energy transition: Future fuels and future emissions,” Journal of Marine Science and Application, vol. 22, pp. 681-692, 2023.
[https://doi.org/10.1007/s11804-023-00369-z]
-
K. Yang, Z. Wang, K. Zhang, D. Wang, F. Xie, Y. Xu, and K. Yang, “Impact of natural gas injection timing on the combustion and emissions performance of a dual-direct-injection diesel/natural gas engine,” Energy, vol. 270, 126813, 2023.
[https://doi.org/10.1016/j.energy.2023.126813]
-
J. D. Ampah, X. Liu, X. Sun, X. Pan, L. Xu, C. Jin, T. Sun, Z. Geng, S. Afrane, and H. Liu, “Study on characteristics of marine heavy fuel oil and low carbon alcohol blended fuels at different temperatures,” Fuel, vol. 310, 122307, 2022.
[https://doi.org/10.1016/j.fuel.2021.122307]
-
H. Chu, R. Hong, W. Dong, H. Zhang, X. Ma, and L. Chen, “Recent advances in soot formation mechanisms: Oxidation and oxidation-induced fragmentation,” Fuel, vol. 371, 132046, 2024.
[https://doi.org/10.1016/j.fuel.2024.132046]